Greenland Resource Assessment,
North Greenland
(Assessment Unit 7)
Executive Summary
A play-based resource assessment of conventional hydrocarbons has been performed for the on- and offshore areas of North Greenland (Assessment Unit 7, AU7). Two sedimentary basins are present in this area:
• The onshore Neoproterozoic – ?earliest Devonian Franklinian Basin
• The offshore ?Carboniferous – ?Palaeogene Lincoln Sea Basin
The onshore Franklinian Basin succession comprises Neoproterozoic – ?lowermost Devonian sedimentary strata and includes early syn-rift to passive margin deposits of mixed carbonate and siliciclastic facies. The total thickness of the sedimentary succession is up to 8 km. The sedimentary succession is well exposed in a homoclinal platform environment in much of northern Greenland. The northern exposures are, however, deformed by the Ellesmerian orogenic structures which possibly are reactivated during the Eurekan orogeny, resulting in the development of a fold and thrust belt. Palaeo-heat-flow indicators show that temperatures increase to the north, where much of the basin is over-mature. Common oil seeps including an exposed Silurian palaeo-oilfield testifies to the petroleum potential of the basin.
Two plays, a Cambro-Ordovician and a Silurian, have been evaluated. Although several formations have good source potential, the general shallow burial depth, with large parts of the succession being exposed in vast areas, the lack of structuring in areas with a mature source rock the exploration potential of the Franklinian Basin is prove to be very limited and hence no volume calculations have been made.
The Lincoln Sea Basin is located in the offshore areas north of the Franklinian Basin. Apart from airborne gravity and magnetic data very little geological and geophysical data are available from the offshore areas, but a few scattered refraction lines and seismic drift lines suggests the presence of a deep sedimentary basin. This basin is part of a major rift complex in the high Arctic and appears to be comparable in width and depth to the Sverdrup Basin of the Canadian Arctic Islands. A reconstruction of the Greenland, North American and European plates suggests that the Wandel Sea nnBasin, the Central Spitsbergen Basin, the Lincoln Sea Basin and the Sverdrup Basin were connected before the onset of seafloor spreading in the Palaeogene. The Triassic onshore successions throughout the Arctic basins show many similarities and the stratigraphy of the Lincoln Sea Basin is modelled in analogy to the Sverdrup, Svalbard and Wandel Sea Basins.
The total thickness of the sedimentary succession in the Lincoln Basin is estimated to be up to 12 km thick.
Due to the lack of seismic data across the Lincoln Sea Basin, no leads have been mapped. Instead, prospective plays, trap size and trap density are assumed to be the same as in the analogue Sverdrup Basin in Arctic Canada. Based on this assumption two prospective plays are evaluated, including a Middle Triassic to earliest Jurassic Play and a Late Jurassic to earliest Cretaceous Play.
Due to the general lack of knowledge and anticipated uplift and deformation during the Eurekan Orogeny of the area the exploration potential of the Lincoln Sea Basin carries a very high risk and consequently the risked volumes are low. The calculated mean risked recoverable volumes of the Lincoln Sea Basin is 800 MMBOE.
Because of the remoteness of the area and the harsh physical conditions Assessment Unit 7 has never been target for oil and gas exploration.
The assessment results presented are based on all available data collected and interpreted by GEUS and other academic institutions and reflect the state of geologic knowledge of the offshore and onshore areas in North Greenland at the time of study. Any future data acquisition, drilling and evaluation of the petroleum systems and plays based on new data within this area will add enormously to the geologic knowledge and will therefore lead to a refinement of these assessment results.
The assessment was carried out using the Player® GIS software provided by GIS-pax. The methodology and results of the assessment have been quality controlled by the Norwegian Petroleum Directorate and GIS-pax.
The results of this study are reported in an extensive ArcGIS project supported by a comprehensive slide package which includes six presentations, reflecting the workflow of the project, including:
1. Introduction
2. Database
3. Structural and Stratigraphic Framework and petroleum plays of the Franklinian Basin
4. Structural and Stratigraphic Framework and petroleum plays of the Lincoln Sea Basin
5. Petroleum Systems – Source Rock Assessment
6. Basin Modelling
7. Play Analysis
8. Yet-to-Find Assessment
The Player and ArcGIS projects together with the slide pack are available for the petroleum exploration industry at no cost and can be downloaded from the project website.
Introduction
The AU7 Resource Assessment (North Greenland) is the last of seven assessments of the “Whole-of-Greenland Petroleum Resource Assessment Project” covering the entire Greenland continental shelf and adjacent onshore basins (Fig. 1). The aim of the project is to provide an estimate of a quantitative, play-based Yet-to-Find potential of conventional hydrocarbons on the Greenland continental shelf. The project was originally initiated to help the Greenland authorities and politicians in strategic decision making and in planning of future natural resource administration.
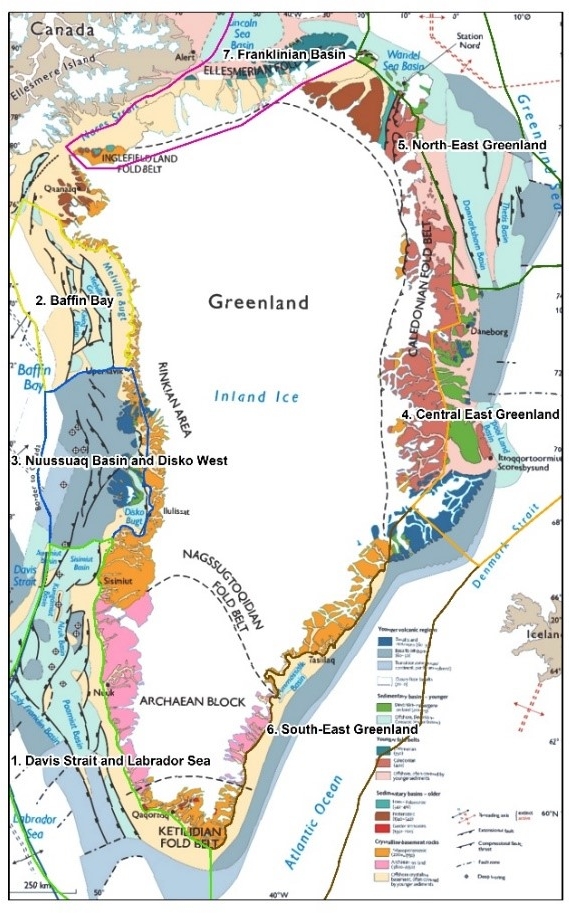
Figure 1: Geological map of Greenland with the seven assessment units indicated.
In addition, it should identify potential needs for further geological and geophysical investigations. When oil and gas exploration was suspended in 2021, it was decided to finalize the project to have a historical document for the future, that examines the oil and gas potential in this part of the Arctic, based on all available academic and industry data.
The project is mainly sponsored by NUNAOIL and is carried out as a collaboration between GEUS, NUNAOIL and the Government of Greenland, and is hosted by GEUS. The work is based on all existing data provided by the industry, GEUS, NUNAOIL and the Greenland Government. Furthermore, it includes a basin modelling study of the Lincoln Sea Basin carried out in-house at GEUS. The results have been reported as the work on the individual assessment units were finalized, and the present report is thus the last of a total of seven reports published as part of the Greenland Resource Assessment.
A hydrocarbon resource assessment North Greenland has previously been performed as part of the U.S. Geological Survey (USGS) Circum-Arctic Resource Program (Gautier & Moore 2008 ) including the Franklinian Shelf province in Arctic Canada and North Greenland (Tennyson & Pitman 2008) and the Lincoln Basin by Sørensen et al. (2011). In the study by Tennyson & Pitman (2008) the product of the assigned probabilities for charge, reservoir rock and timing used by USGS for the Franklinian Basin were less than the minimum probability required for quantitative assessment by USGS and no volumes were calculated. The Lincoln Sea Basin offshore North Greenland was not part of the USGS 2007 appraisal programme.
Based on two long wide-angle seismic reflection/refraction profiles and a short vertical incident reflection profile (Jackson et al. 2010) suggested the presence of Palaeozoic to Mesozoic age strata, in the ice covered offshore areas off North Greenland. Based on analogues from the Mesozoic strata in the Sverdrup and Svalbard Basins, (Sørensen et al. 2011) carried out a resource assessment using on the same assessment methodology as the USGS 2007 assessment and they came up with a Pmean of 1135 MMBOE including both the Canadian and Greenlandic parts of the basin.
Database
The database for the Franklinian Basin includes world-class outcrops of the Neoproterozoic and Palaeozoic succession and cores from 11 shallow boreholes drilled by the Geological Survey of Greenland (GGU) in the mid-1980s (Fig. 2). The offshore Lincoln Sea Basin is covered by a few single reflection seismic drift lines (Funck et al. 2006; Jackson et al. 2010; Kristoffersen et al. 2021). Other geophysical data includes gravity and magnetic anomaly data. Carboniferous-Paleogene analogue basins include the Sverdrup Basin in Arctic Canada, the Wandel Sea Basin in easternmost North Greenland, and the Svalbard basins in the Arctic Ocean. A large number of scientific publications are available from these analogue basins..
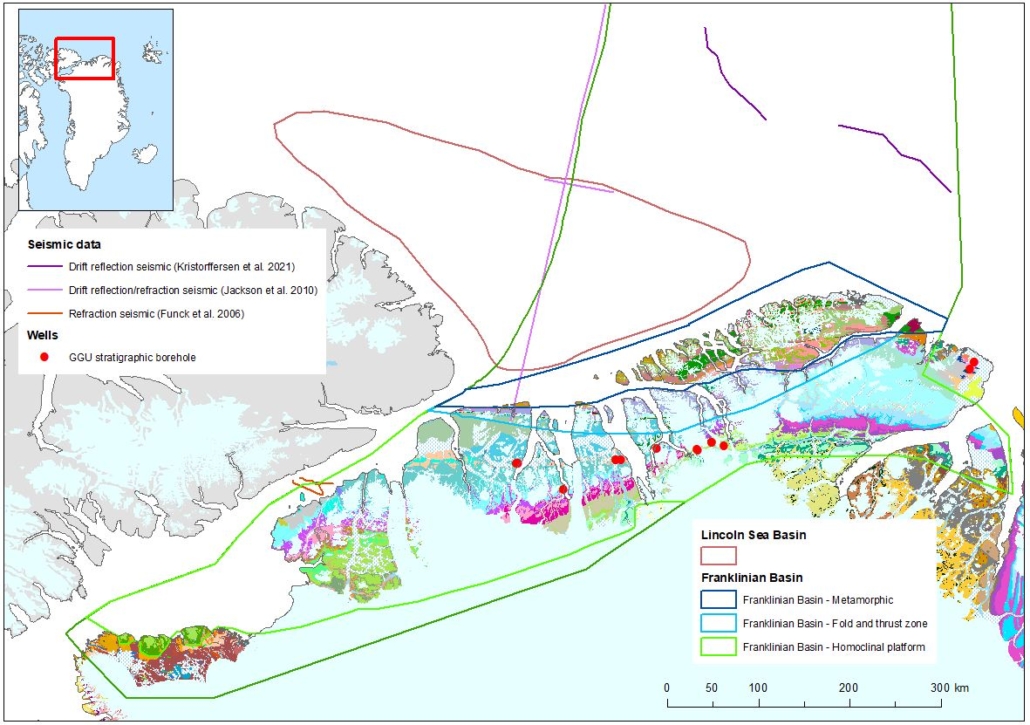
Figure 2 – Seismic and well database for AU7. Geological background map shows Franklinian Basin geology within AU7.
Franklinian Basin
The latest Neoprotozoic to ?earliest Devonian Franklinian Basin extends east–west across North Greenland and Arctic Canada, with a total exposed length of 2500 km (Fig. 2). Exposures in North Greenland are world-class with only a thin cover of Quaternary surficial deposits and ice sheets. Any overlying Devonian deposits have been completely removed, but conodont alteration data (CAI) and organic maturity data show that any possible cover succession south of the Ellesmerian orogenic belt has been thin (~1 km) in contrast to the thick Devonian foreland basin succession present in Arctic Canada (Surlyk 1991; Higgins et al. 1991).
In the eastern part of North Greenland (Assessment Unit 4) the Franklinian Basin deposits are unconformably overlain by Carboniferous–Palaeogene sedimentary rocks of the Wandel Sea Basin (Stemmerik & Håkansson 1991; Håkansson et al. 1991).
Structural and Stratigraphic Framework and Petroleum Plays
The Franklinian Basin succession records a complete Wilson cycle: rift – break-up – passive margin – ocean closure/orogeny which has been divided into seven basin evolution stages (Figs 3, 4; see Higgins et al. 1991; Ineson & Peel 1997; Hopper & Ineson 2021 for detailed descriptions).
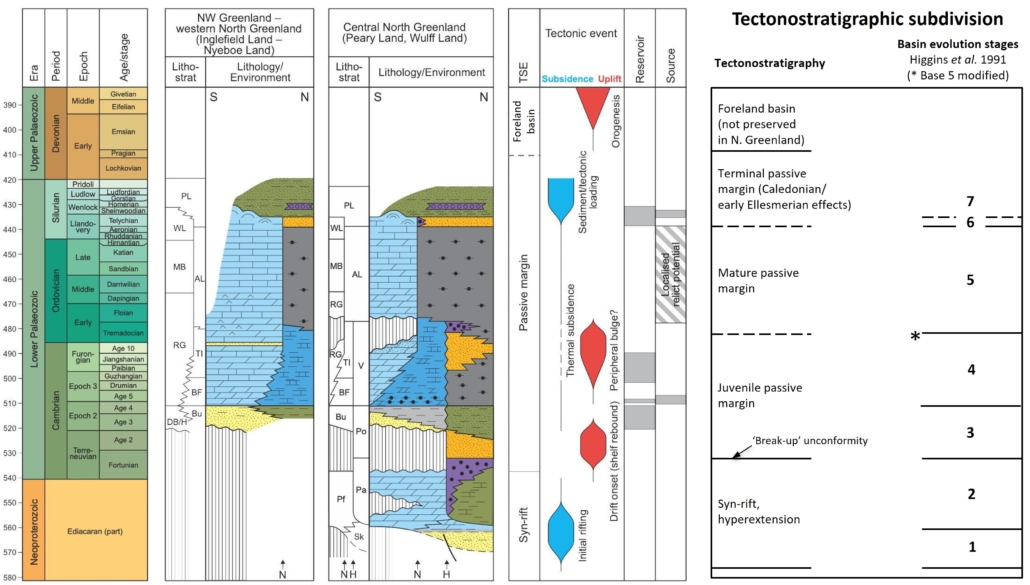
Figure 3 – Tectonostratigraphic chart of the Franklinian Basin showing the distribution of play elements and tectonic events. AL: Amundsen Land Group; BF: Brønlund Fjord Group; BU: Buen Formation; DB/H: Dallas Bugt/Humboldt Formations; H: Harder Fjord fault zone; HF: Hagen Fjord Group; IF: Independence Fjord Group; MB: Morris Bugt Group; N: Navarana Fjord escarpment; Pa: Paradisfjeld Group; Pf: Portfjeld Formation; PL: Peary Land Group; Po: Polkorridoren Group; RG: Ryder Gletscher Group; Sk: Skagen Group; TI: Tavsens Iskappe Group; V: Vølvedal Group; WL: Washington Land Group. Modified from Hopper & Ineson (2021).
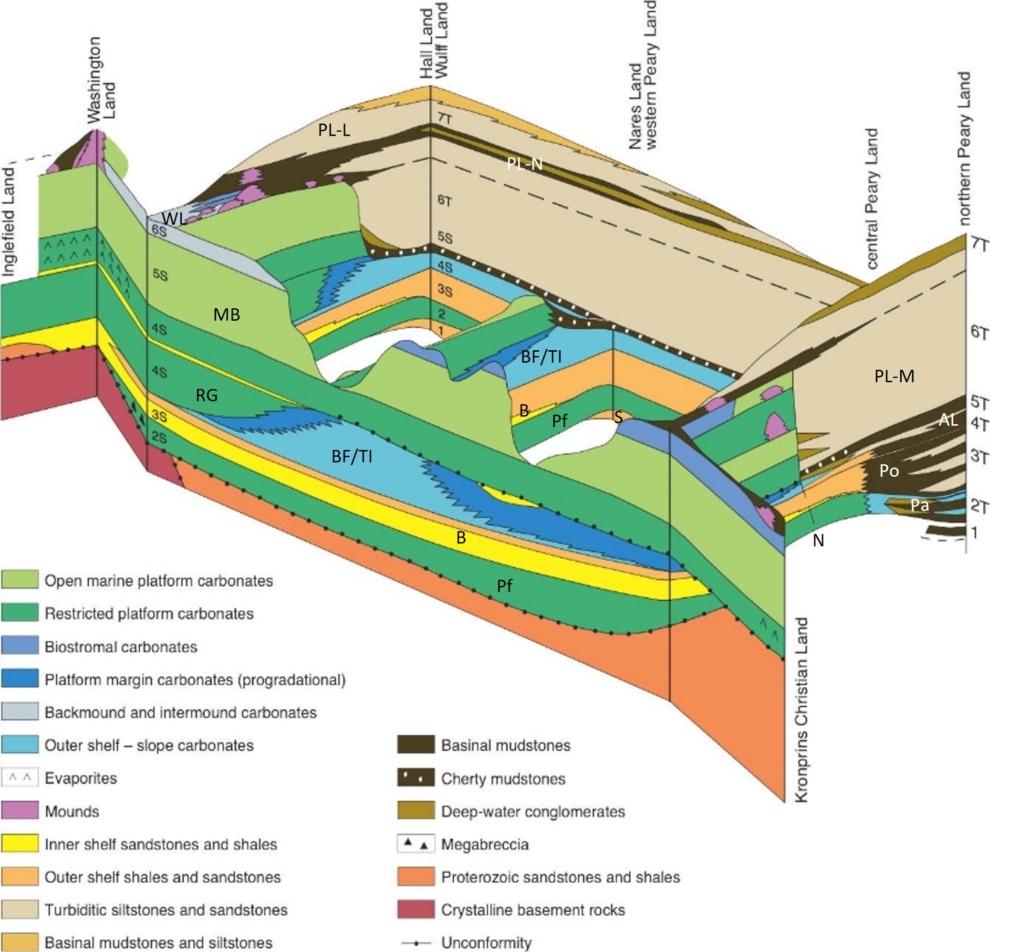
Figure 4 – Fence diagram showing the interpreted relationships of shelf, slope and trough deposits of varius stages (numbered S for shelf, T for trough) in the evolution of the Franklinian Basin from Inglefield Land in the west to Kronprins Christian Land in the east. PL-M: Merqujôq Fm; PL-N: Nordkronen Fm ; PL-L: Lauge Koch Land Fm – other abbreations as Fig 2. Modified from Higgins et al. (1991).
Stage 1: Syn-rift siliciclastic deposition
The syn-rift succession is divided into two stages (Higgins et al. 1991). Stage 1 comprises a varied, >500 m thick succession of siliciclastic sediments and subordinate carbonates of the Skagen Group (Fig. 3) deposited in offshore shelf and shallow-water nearshore environments. It is only exposed north of the Navarana Fjord escarpment in the fold and thrust region of the Franklinian Basin (Figs 2–4). The deposits are not well known but precede the development of discrete shelf and deep-water basin settings established during Stage 2. The Skagen Group is exposed in isolated major anticlines (Fig. 5). The deposits are slightly metamorphosed and consequently have no exploration potential.
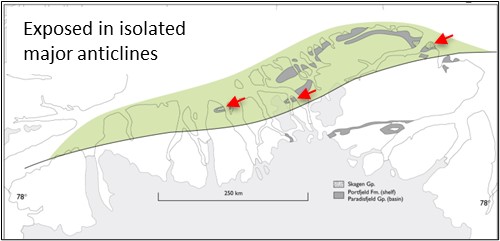
Figure 5 – Distribution of Stage 1 syn-rift siliciclastic and carbonate sediments. The strata are mainly exposed in isolated major anticlines (red arrows). J.R. Ineson, unpublished.
Stage 2: Syn-rift carbonate shelf/deep-water basin development
The second syn-rift stage is marked by the development of a carbonate shelf represented by the Portfjeld Formation, a succession of ramp and shallow-water platform carbonates ranging from 200 to 300 m in thickness in the southern exposures to 700 m in the north. It conformably overlies the Skagen Group in the north and rests unconformably on basement in the south, indicating an overall southward transgression and expansion of the depocentre between Stages 1 and 2 (Figs 3, 6). Farther north, partly coeval deeper-water deposits are represented by the Paradisfjeld Group, which includes siliciclastic and carbonate mudstones, turbidites, and resedimented conglomerates. The Paradisfjeld Group marks the early stages of a deep-water basin development with the formation of an abrupt shelf edge governed by the position of the Harder Fjord fault zone (Fig. 3). The deposits of this stage lack good reservoirs and as well as source rocks, and are not considered to have any exploration potential.
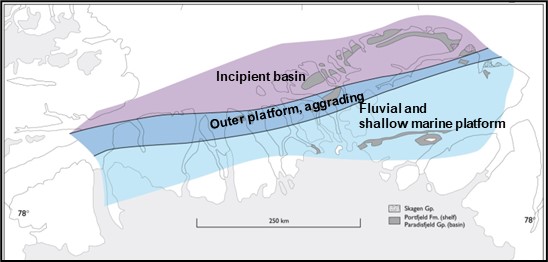
Figure 6 – Distribution of Stage 2 syn-rift carbonates. The shelf consists of ramp and shallow-water platform carbonates. and tThe basin margin of carbonate slope aprons become shaly shaling towards the north. J.R. Ineson, unpublished.
Stage 3: Early Cambrian juvenile passive margin
The change from syn-rift to passive margin deposition is marked by uplift and erosion of the shelf succession resulting in the development of an unconformity between the Portfjeld and Buen Formations (Stage 3), probably reflecting break-up and the onset of sea-floorseafloor spreading to the north. Associated with uplift is deposition of shelf megabreccias and karstification of the Portfjeld carbonates. During Stage 3 a well-defined slope developed governed by the position of the Harder Fjord fault zone (Figs 3, 7) with deposition of true deep-water sediment to the north (Polkorridoren Group). The shelf deposits are represented by the Buen Formation, a succession of mainly marine sandstones and mudstones. The proportion of mudstone increases northwards as does the overall thickness (up to 700 m) before giving way to the slope and deep-water basinal deposits of the Polkorridoren Group. The latter is a 2–3 km thick succession of sandstone turbidites and mudstones reflecting a dramatic increase in subsidence to the north.
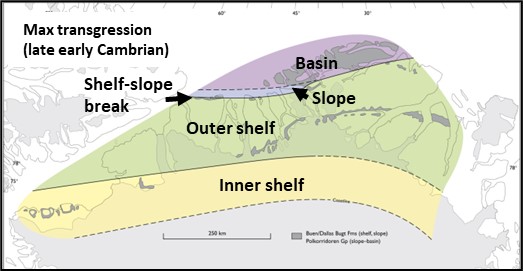
Figure 7 – Distribution of Stage 3 deposits. A broad inner–outer siliciclastic shelf developed during this stage with a well-defined transition into a deep-water turbidite basin to the north. The shelf-slope break follows the Navarana Fjord escarpment which also is the approximate southern boundary of the fold and thrust zone of the Ellesmerian orogen to the north. J.R. Ineson, unpublished.
Play 13B2: Early Cambrian fluvial – shallow shelf sandstone play
The Early Cambrian shelf sandstones of the lower Buen Formation provide good reservoir and top seal is provided by outer shelf shales of the upper Buen Formation and the overlying carbonate mudstones and shales of the Brønlund Fjord Group. Long-distance migration of hydrocarbons from either Cambrian or Ordovician marine organic-rich shales is anticipated for this play (Fig. 8).
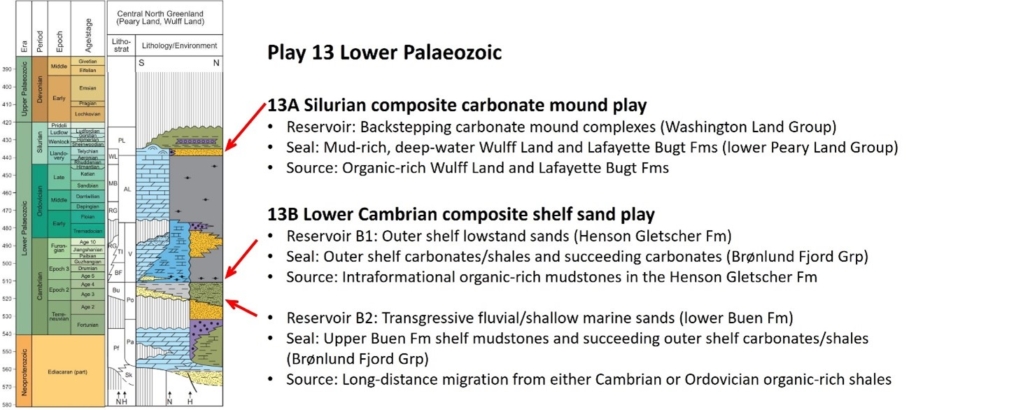
Figure 8 – Early Palaeozoic plays of the Franklinian Basin. See Fig. 2 for legend.
Stage 4: Cambrian–Middle Ordovician passive margin
The late early Cambrian – Middle Ordovician marks a return to a carbonate-dominated shelf to the south with a generally sediment-starved deep-water basin in the north (Figs 3, 4, 9). The shelf developed as a progradational platform – deep shelf system in the east, represented by the Brønlund Fjord and Tavsens Iskappe Groups, and a uniformly subsiding aggradational shelf in the west, represented by the Ryder Gletscher Group (Ineson & Peel 1997). The equivalent deep-water deposits in the eastern region are represented by the Vølvedal Group, which includes thick sandstone turbidite units intercalated with dark cherty mudstones.
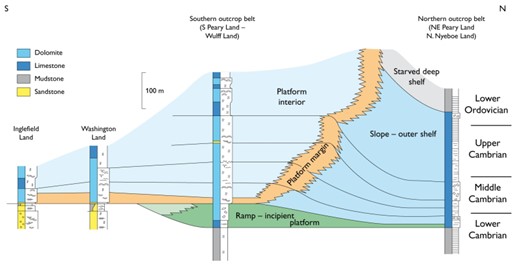
Figure 9 – Geosection showing the facies distribution during Cambrian – earliest Ordovician time (Stage 4). J. Ineson, unpublished??.
Play 13B2: Middle Cambrian – Ordovician shelf sandstone play
Middle Cambrian shelf sandstones of the Henson Gletscher Formation provides good reservoirs, and top seal is provided by outer shelf carbonates and shales of the Brønlund Fjord Group. Well-documented organic-rich carbonate mudstone units within the Henson Gletscher Formation provide source for petroleum (Fig. 8).
Stage 5: Middle Ordovician – earliest Silurian passive margin
Stage 5 is marked by major backstepping of the shelf margin to the position of the Navarana Fjord escarpment. The escarpment defined the northern margin of an aggradational carbonate platform that accreted vertically, anchored by differential subsidence, forming the scarp (Figs 3, 4).
The carbonate platform is represented by the Late Ordovician Morris Bugt Group, which consists of a number of stratigraphic units of uniform thickness, indicating steady subsidence over the entire platform area. The slope region (the outer-shelf zone of the previous stage) and the deep-water basin were sediment starved and are represented by the Amundsen Land Group. The slope succession is thin (<200 m) and dominated by dark cherty mudstones with localized redeposited carbonate beds; the deep-water basin succession is thicker (up to 500 m) but similar in facies development, albeit with thick redeposited carbonate conglomerate units at certain levels. An influx of thin-bedded silty turbidites in the uppermost Amundsen Land Group heralded the onset of sand-rich turbidite sedimentation in Stage 6.
Althoug the Aleqatsiaq Fjord Formation shows staining in Washington Land – possibly from an intraformational source interval – this succession lacks good reservoirs and is not considered to have any exploration potential.
Stage 6: Early Silurian terminal passive margin (Caledonian and early Ellesmerian effects)
This stage is marked by major changes in the depositional systems, both on the shelf and in the deep-water basin (Figs 3, 4). The Silurian Merqujôq Formation of the lower Peary Land Group is a thick (up to 2800 m) succession of sandstone turbidites that is widespread in North Greenland. Rapid infill of the deep-water basin and the consequent sedimentary loading may have resulted in down-flexure of the shelf, increasing the overall rate of basinal subsidence. Current directions are consistently westwards, suggesting that the rising Caledonian mountains to the east were the source of the sedimentary influx.
Carbonate deposition (Washington Land Group) continued on the shelf, but the increasing subsidence rate forced the shallow-water shelf depositional belts to migrate southwards as initial drowning of the platform began. Large carbonate mounds developed along the outer rim of the shelf.
Stage 7: Late Silurian terminal passive margin (Caledonian and early Ellesmerian effects)
By the Late Silurian the shelf was drowned and inundated by hemipelagic mudstones and siltstone turbidites that form part of the upper Peary Land Group (Figs 3, 4). Carbonate deposition persisted locally in the mid–late Silurian, forming isolated mound build-ups and pinnacle reef complexes that were probably ultimately overwhelmed in Late Silurian (Ludlow) times. The sandstone turbidite systems of the Peary Land Group continued to fill the axial basin and, towards the top of succession, a major phase of conspicuous chert conglomerate deposition was initiated in the middle Wenlock (mid–late Silurian) sourced from the rising Caledonian mountains in the east. The youngest fine-grained turbiditic sediments in the Franklinian Basin in Greenland of latest Silurian – ?earliest Devonian age are located in western North Greenland.
Play 13A: Silurian carbonate mound play
The huge Silurian composite carbonate mounds of the Washington Land Group provide reservoir and whereas top seal and source is are provided by deep-water organic rich mudstones of the Wulff Land and Lafayette Bugt Formations (upper Peary Land Group; Fig. 8). A large exhumed bitumen-impregnated Silurian reef indicates that this petroleum system has worked (Stemmerik et al. 1997).
Lincoln Sea Basin
The Lincoln Sea Basin is located in shallow water north of the Franklinian Basin in North Greenland and Arctic Canada of which approximately half is in Canadian territorial waters (Figs 2, 10). The basin is defined by very few data including magnetic data (Kovacs 1982; McMillan 1982), a short seismic refraction line (Forsyth et al. 1994), and a combination of gravity and refraction seismic data (Jackson et al. 2010).
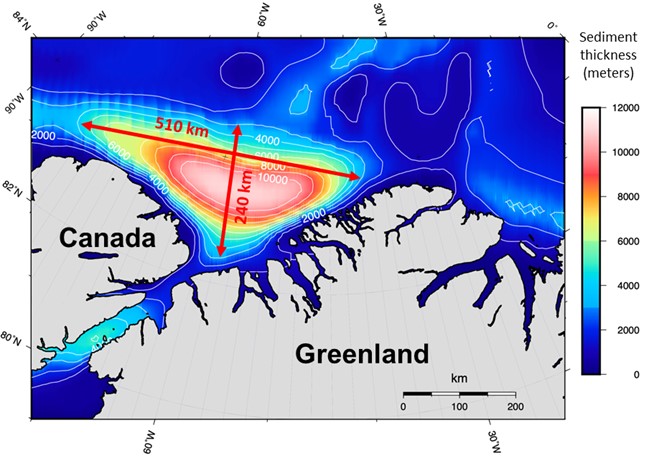
Figure 10 – Thickness map of the Lincoln Sea Basin based on three-dimensional gravity inversion (Lebedeva-Ivanova et al. 2019).
The work by Jackson et al. (2010) showed the presence of a sedimentary basin up to 12 km deep consisting of three layers with velocities of 2.1–2.2, 3.1–3.2 and 4.3–5.2 kms-1. Based on local and regional geological constraints the 2.1–2.2 kms-1 and 3.1 – 3.2 kms-1 sections are correlated with Arctic continental terrace sediment wedges bordering the Canadian Arctic Islands. The underlying 4.3 – 5.2 kms-1 section is correlated with the onshore Sverdrup Basin Palaeozoic to Mesozoic succession in Arctic Canada (Fig. 11).
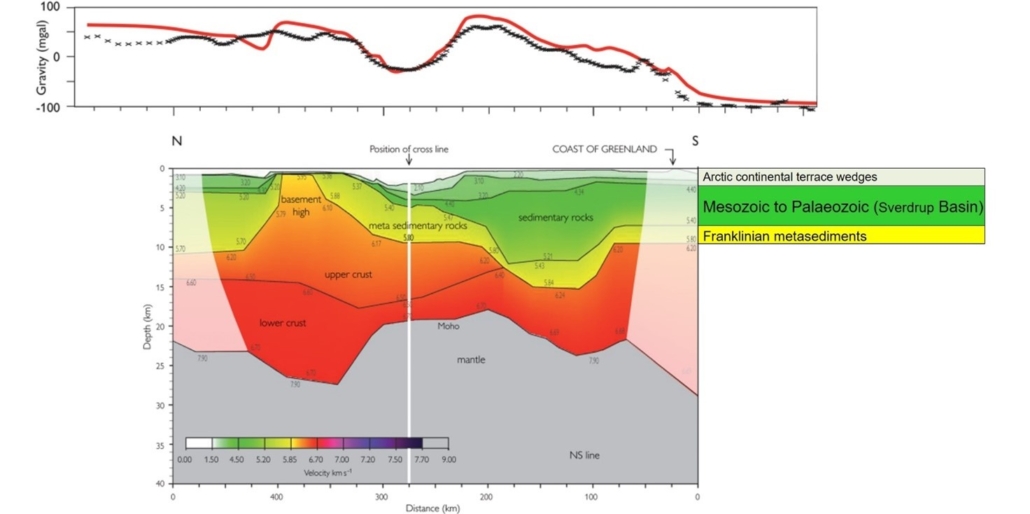
Figure 11 – Velocity model of the LORITA-1 NS line with resulting in modelled gravity response (red) compared with measured gravity (black). See Fig. 2 for location of line. From Jackson et al. (2010).
Stratigraphic Framework and Petroleum Plays
The outline of the basin is not well constrained due to the very limited amount of data available, but for this study the 4 km thickness outline of Lebedeva‐Ivanova et al. (2019) has been used (Fig. 10). Although both the interpreted thickness and size of the Lincoln Sea Basin should be taken with reservation it appears to be comparable to Sverdrup Basin. The stratigraphy of the Lincoln Sea Basin has therefore been suggested to be analogous to the Sverdrup and Svalbard Basins (Sørensen et al. 2011) and the Wandel Sea Basin in northern Greenland described below. Figure 12 shows the tectono-stratigraphy of the Sverdrup and Svalbard Basins. In the Sverdrup and Svalbard Basins three main plays have been proven and are described below as Plays 6, 7 and 8.
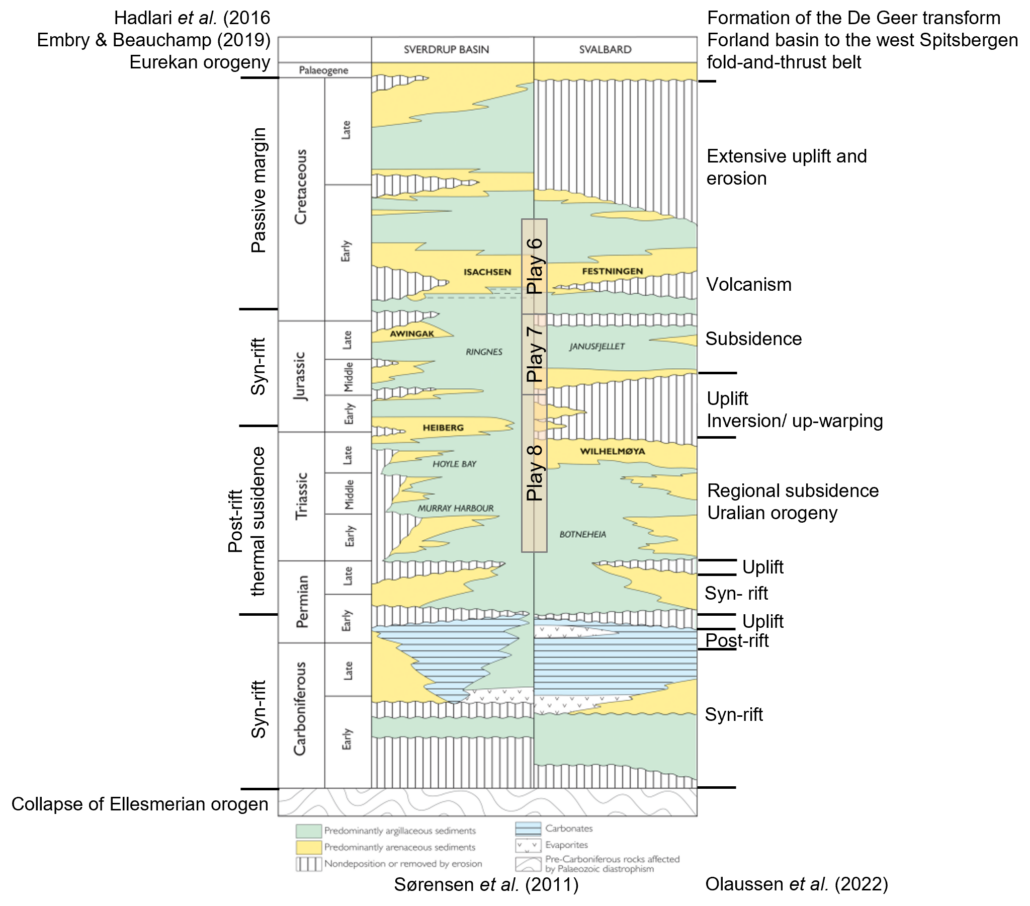
Figure 12 – Tectono-stratigraphy of the Sverdrup and Svalbard Basins. Formation names in bold are proven reservoirs and formation names in italics are main source rock units. Based on Sørensen et al. (2011), Hadlari et al. (2016), Embry & Beauchamp (2019) and Olaussen et al. (2022). Working plays indicated.
Palaeogeography
A structural reconstruction of pre-Eurekan North Greenland is important to establish the palaeogeography for the region and incorporate the geological information from the analogue basins. The dextral shear motion between Greenland and Svalbard from Chron 24 to Chron 21 (Early Eocene) along the de Geer Fault was nearly 200 km and involved crustal extension of 100–150 km north of Spitsbergen (Kristoffersen et al. 2020). The reconstruction of the Greenland and the North American plates relative to Europe and the position of the Lincoln Sea Basin at Chron 21 time (~47 Ma) is shown in Fig. 13.
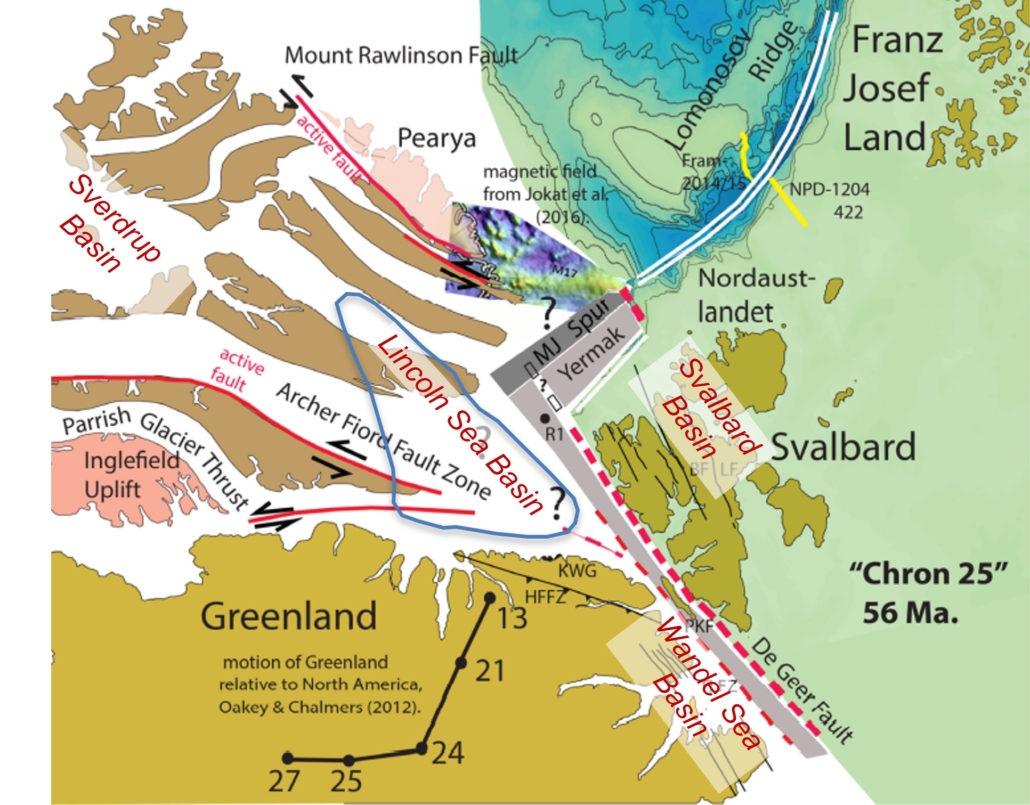
Figure 13 – Reconstruction of Greenland and North American plates relative to Europe at Chron 21 time (47 Ma) after Kristoffersen et al. (2021). Position of Sverdrup, Lincoln Sea, Wandel Sea and Svalbard Basins shown.
Prior to Arctic Ocean sea-floor spreading the Sverdrup, Lincoln Sea, Wandel Sea and Svalbard Basins were probably connected showing an overall similar tectono-stratigraphic evolution following the suture of continental crust during the Late Palaeozoic (Embry 1989; Stemmerik & Worsley 1989; Christie & Dawes 1991). Based on this assumption a series of palaeogeographic maps including the Lincoln Sea Basin have been produced for the most important stratigraphic levels with regards to hydrocarbon play intervals.
Play 8: Late Permian – Triassic Post-rift Thermal Subsidence Play
During the Middle Triassic (Anisian–Landinian) the Arctic and North Greenland basins were characterized by thermal subsidence with deposition of marine organic-rich shelf mudstones under anoxic conditions (Fig. 14). The mudstones provide the most important source rocks in the Sverdrup Basin and Barents Sea and are also known from the Wandel Sea and Svalbard Basins (Embry & Beauchamp 2008; Dallmann et al. 2015; Bjerager et al. in press).
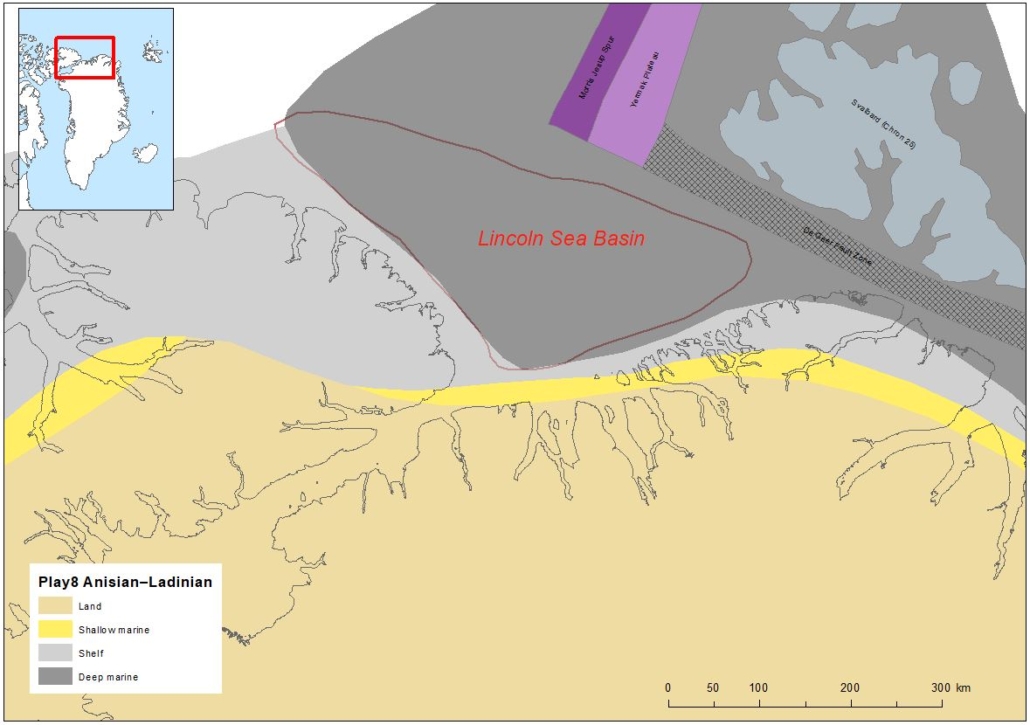
Figure 14 – Middle Triassic (Anisian–Landinian) palaeogeography. During this phase deposition of marine organic-rich mudstones took in the Sverdrup, Wandel Sea and Svalbard Basins, and most likely also the Lincoln Sea Basin. Structural reconstruction from Kristoffersen et al. (2021).
Deposition of most important reservoir sandstones took place during regional subsidence in the latest Triassic – earliest Jurassic (Norian–Rhaetian). During this phase a huge delta system prograding towards west from the Uralian Orogen in Siberia was located in the Barents Sea/Svalbard. Deltaic and paralic deposition took place in the Svalbard Basins whereas the Wandel Sea and Sverdrup Basins – and therefore probably also the Lincoln Sea Basin – were dominated by paralic sedimentation (Fig. 15; Embry & Beauchamp 2008; Dallmann et al. 2015; Bjerager et al. in press).
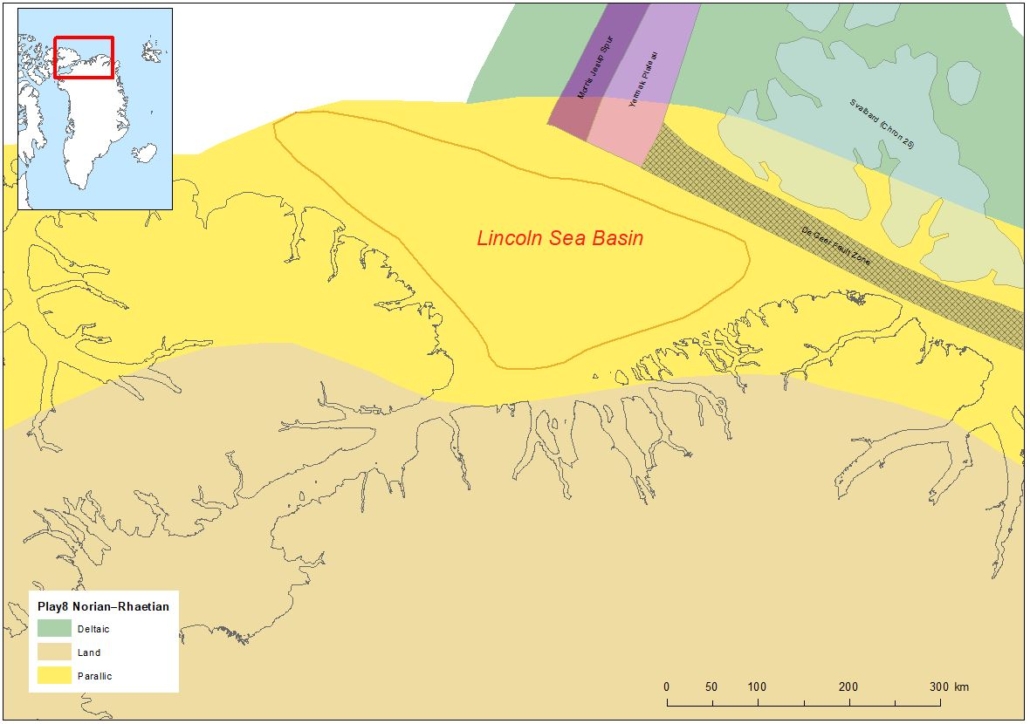
Figure 15 – Latest Triassic – earliest Jurassic palaeogeography. During this phase deposition of paralic and deltaic sandstones took place in the Sverdrup, Wandel Sea and Svalbard basins, and most likely also in the Lincoln Sea Basin. Structural reconstruction from Kristoffersen et al. (2021).
Play 7: Jurassic Rift Play
A new rift phase took place during the Jurassic and several siliciclastic reservoirs-seal pairs composed of shallow marine sandstones and shelf mudstones were deposited in the analogue basins. The play is proven in the Sverdrup Basin where the most important reservoir unit is the Awingak Formation (Fig. 12). During the Late Jurassic deposition of marine organic-rich mudstones under anoxic conditions gave rise to wide-spread and important source rocks recognized in the Sverdrup Basin (Ringnes Formation) and Barents Sea and also known from the Svalbard and Wandel Sea Basins (Figs 12, 16; Dypvik et al. 2002; Embry & Beauchamp 2008; Dallmann et al, 2015; Bjerager et al. in press).
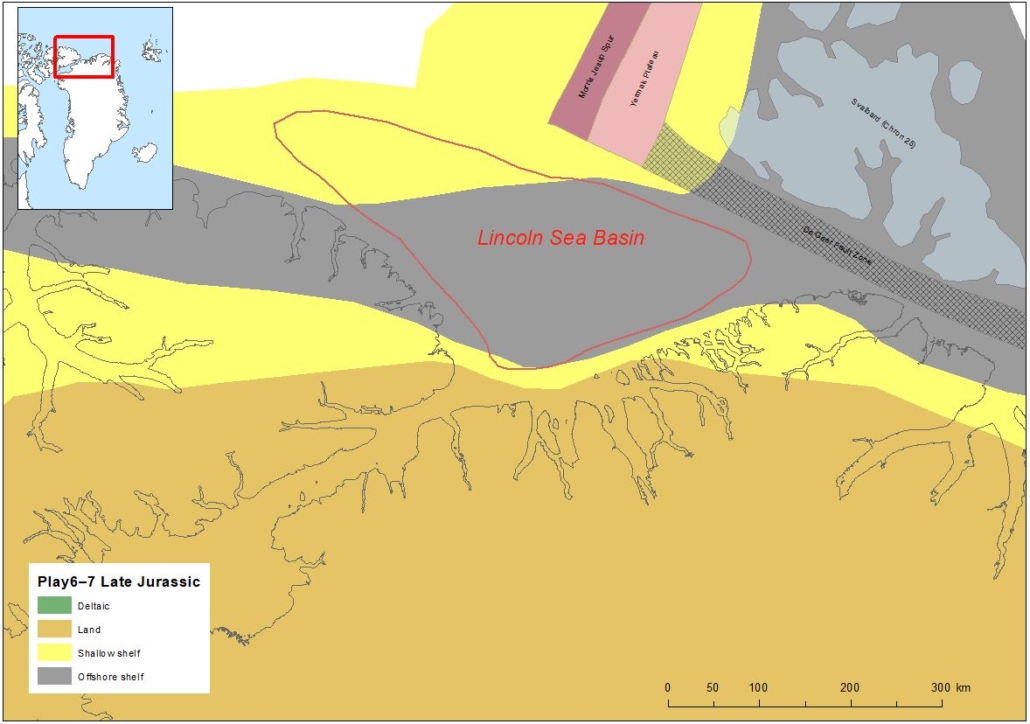
Figure 16 – Late Jurassic palaeogeography. During this phase marine organic-rich mudstones were deposited under anoxic conditions in the Sverdrup, Wandel Sea and Svalbard Basins and these conditions probably also prevailed int the Lincoln Sea Basin. Along the basin shallow marine sandstones were deposited. Structural reconstruction from Kristoffersen et al. (2021).
Play 6: Cretaceous Passive Margin Play
In the analogue Sverdrup Basin this stage is characterized by shallow marine sicliciclastic deposits and an important reservoir in the shallow marine Isachsen Formation (Fig. 12). Here the organic-rich Late Jurassic marine mudstones of the Ringnes Formationof Play 7 also provide the source rock.
Rifting continued into the Early Cretaceous resulting in increased subsidence of the Sverdrup Basin where shallow marine sicliciclasticsiliciclastic deposits of the Isachsen Formation provide an important reservoir (Fig. 12). A thick succession of basaltic volcanic rocks were extruded on Ellesmere Island and Axel Heiberg Island in Arctic Canada and has been related to the occurrence of a hotspot located north of the Sverdrup Basin (Fig. 16; Embry & Beauchamp 2008). Shallow marine sandstones and shelf mudstones were deposited in North Greenland and Svalbard basins (Dypvik et al. 2002; Dallmann et al. 2015), but it is uncertain if shallow marine conditions also prevailed in the Lincoln Sea Basin or if the basin was part of the volcanic province known from Arctic Canada (Fig. 17).
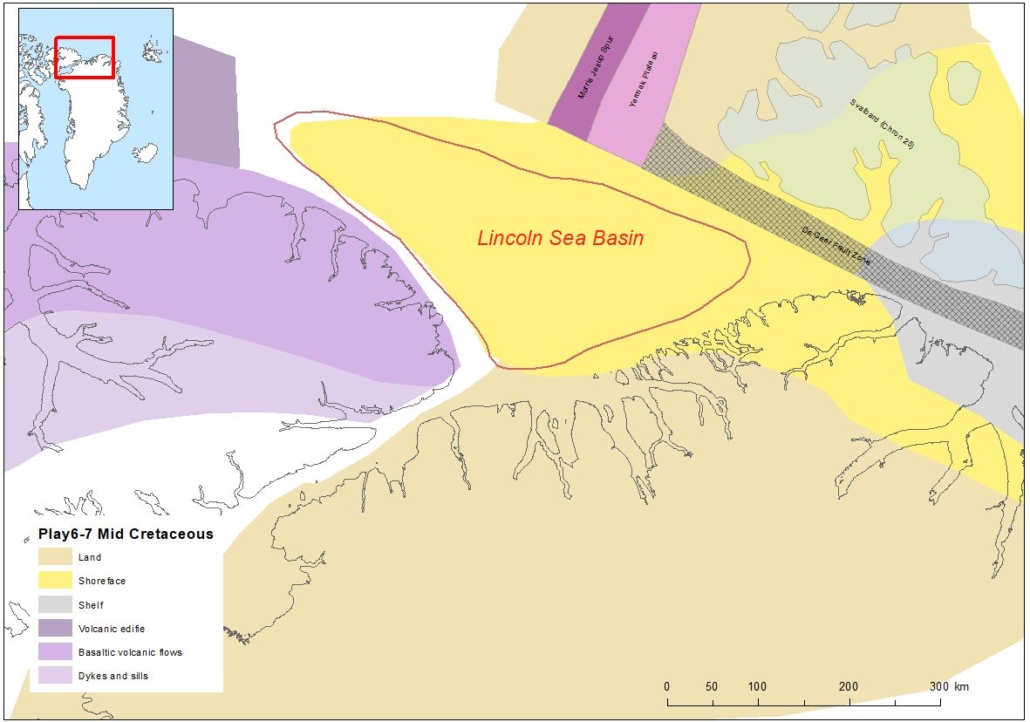
Figure 17 – Late Cretaceous palaeogeography. Thick successions of volcanic rocks were extruded on Ellesmere Island and Axel Heiberg Island are related to a hot spot north of the Sverdrup Basin. Structural reconstruction from Kristoffersen et al. (2021).
Trapping mechanism: Carboniferous salt and halokinesis
The main trapping mechanism for plays 6, 7 and 8 is anticipated to be associated with salt domes as in the analogue Sverdrup Basin. Widespread carbonate and evaporite sedimentation related to Carboniferous rifting has been recorded in the Canadian Arctic Islands, North Greenland (Sortebakker Formation; Stemmerik & Håkansson 1991; Dalhoff & Stemmerik 2000) and Svalbard (Nøttvedt et al. 1993). Deposition of Carboniferous – Early Permian evaporites in the deeper part of the basin is critical for the development of salt domes.
The main phase of halokinesis took place during the Eocene Eurekan orogenesis (Embry & Beauchamp 2019) which resulted from major changes in the plate motion accompanied with northward drift and counter-clockwise rotation of Greenland relative to North America during opening of the Labrador Sea – Baffin Bay Seaway and the North Atlantic (e.g. Oakey & Chalmers 2012). In the eastern part of the Sverdrup Basin this orogeny was severe and appears to have resulted in leakage of hydrocarbons from Mesozoic traps. The Eurekan orogenesis is also expected to have impacted the Lincoln Sea Basin, but to what extent it has caused breaching of the seal of potential traps is unknown. All three plays are believed to have Eurekan age anticlines associated with halokinesis as trap, but other structural traps could be fault-bounded 3-way dip closures associated with Carboniferous or Jurassic rifting. In addition, reefs and prominent unconformities could act as trapping mechanisms.
Petroleum Systems
Onshore indications of viable petroleum systems in AU7 include a number of locations that show bitumen and oil stains ( Fig. 18; Christiansen & Bojesen-Koefoed 2021).
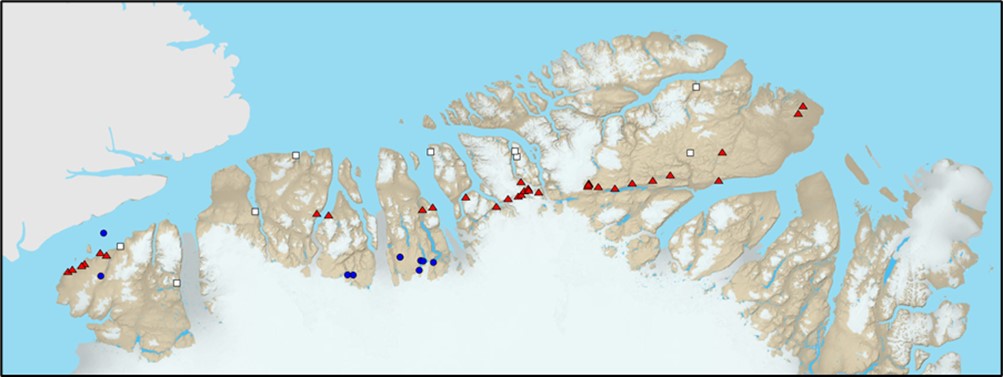
Figure 18 – Distribution of petroleum seeps and stains in North Greenland. Red triangles: petroleum seepage and stains associated with source rock. Blue circles: petroleum seeps and stains, migrated. White squares: solid bitumen associated with mineral occurrences. From Christiansen & Bojesen-Koefoed (2021).
Large parts of the Franklinian Basin fall within the thermally overmature to low-grade metamorphic window and the oil-window maturity is restricted to a very narrow zone (Fig. 19). The southern part of the Franklinian Basin is termally immature.
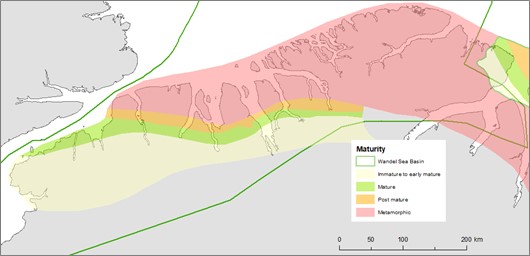
Figure 19 – Simplified thermal maturity map of North Greenland based on all available data. The green polygons indicate areas where source rocks are oil mature. From Christiansen (1989).
Bitumen and oil stains associated with locally matured source rocks in immediate vicinity includes the Cambrian Henderson Gletscher Formation, the Ordovician Aleqatsiaq Fjord Formation and the Silurian Lafayette Bugt and Wulff Land Formations (Figs 3, 8; Christiansen & Bojesen-Koefoed 2021).
A number of locations show bitumen that cannot be readily linked to a local source (blue circles in Fig.18), and long-distance migration (up to approximately 100 km) must be invoked to explain prominent presence of bitumen and seepage in sandstones and carbonates far from mature sources within regions of thermal immaturity. Details on the Cambrian, Ordovician and Silurian source rocks are published in Christiansen et al. (1987) and Christiansen (1989).
The very limited data from the the Lincoln Sea Basin does not include any information that could indicate a working petroleum system. Possible source rock information is therefore only available from the analogue basins.
From the Sverdrup and Svalbard Basins and from the Barents Sea, Carboniferous, Permian, Triassic, Jurassic source rocks are known and a Cretaceous source rock is furthermore present in the Sverdrup Basin (see van Koeverden et al. 2011; Sørensen et al. 2011; Berglar et al. 2022 and Wesenlund et al. 2022 and references therein).
However, only the Middle Triassic and Late Jurassic source rocks are of significance and wide-spread in the Arctic region and based on analogy these are most likely source rocks in the Lincoln Sea Basin if a Mesozoic succession is present. Oil stains have also been reported from the Middle Triassic Dunken Formation (Wandel Sea Basin) in north-eastern Greenland (Christiansen & Bojesen-Koefoed 2021).
Table 1 lists the parameters used for the modelling of the potential source rocks in the Lincoln Sea Basin based on the regional analogues.
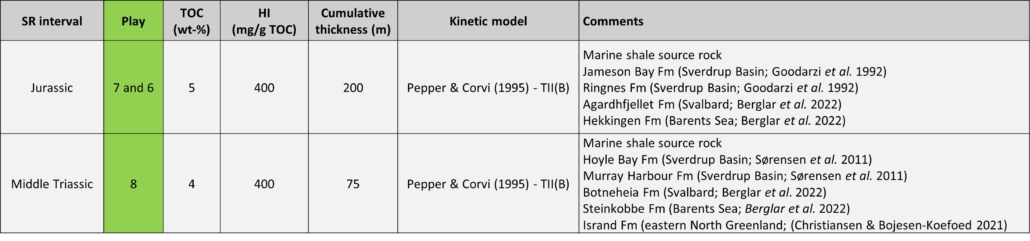
Table 1 – Source rock intervals identified for Lincoln Sea Basin showing values selected for the basin modelling study.
Basin Modelling
As part of their resource assessesment study of the Lincoln Sea Basin Sørensen et al. (2011) a 1-D basin modelling study was performed on two pseudowells in the Sverdrup Basin testing two heat flow scenarios as only variables with an assumed Cenozoic uplift of 1000 m.
In order to evaluate a variety of maturity models for the Lincoln Sea Basin a basin modelling study with a variety of scenarios has been carried out in order to evaluate:
1. Impact of Cenozoic uplift on maturity history,
2. Impact of Late Jurassic and Early Paleocene rifting (as response to formation of the mid-oceanic Gakkel Ridge) on maturition history,
3. Impact of stratigraphic thickness variations on maturation history, and
4. The optimal thickness scenario to ensure that the two most important source rock intervals are in the main oil window (0.70-1.0% Ro) at present.
The study shows that the various Cenozoic uplift scenarios (0, 1000 and 1500 m) have no significant impact on maturition history.
Incorporating a Late Jurassic rifting scenario shows that the Triassic source rock enters the main oil window (0.70% Ro) in the Late Jurassic, prior to the rifting event, and enters the dry gas window (2.0% Ro) during Late Jurassic rifting. The Jurassic source rock enters the main oil window (0.70% Ro) in the Late Cretaceous and remains there until present.
The Early Paleocene rifting scenario shows that the Triassic source rock enters main oil window (0.7% Ro) in the Late Jurassic and and the dry gas window (2.0% Ro) during the mid-Cretaceous. The Jurassic source rock enters the main oil window (0.70% Ro) in the mid-Cretaceous and the late oil window (1.00% Ro) during xxx rifting where it remains until present.
In order for both the Middle Triassic and Upper Jurassic source rocks to be in the main oil window at present day modelling has to be carried out with slightly thinner stratigraphic packages than the Sverdrup Basin PW1 pseudowell of Sørensen et al. (2011). In such a scenario, the Middle Triassic source rock enters the main oil window (0.70 %Ro) in the early Late Cretaceous and the Late Jurassic source rock enters the main oil window in the Late Paleocene (Fig. 20A). The Petroleum System Chart in Figure 20B shows that in the present scenario all play elements are in place (reservoir, seal, trap, source rock) prior to migration from the two source rocks.
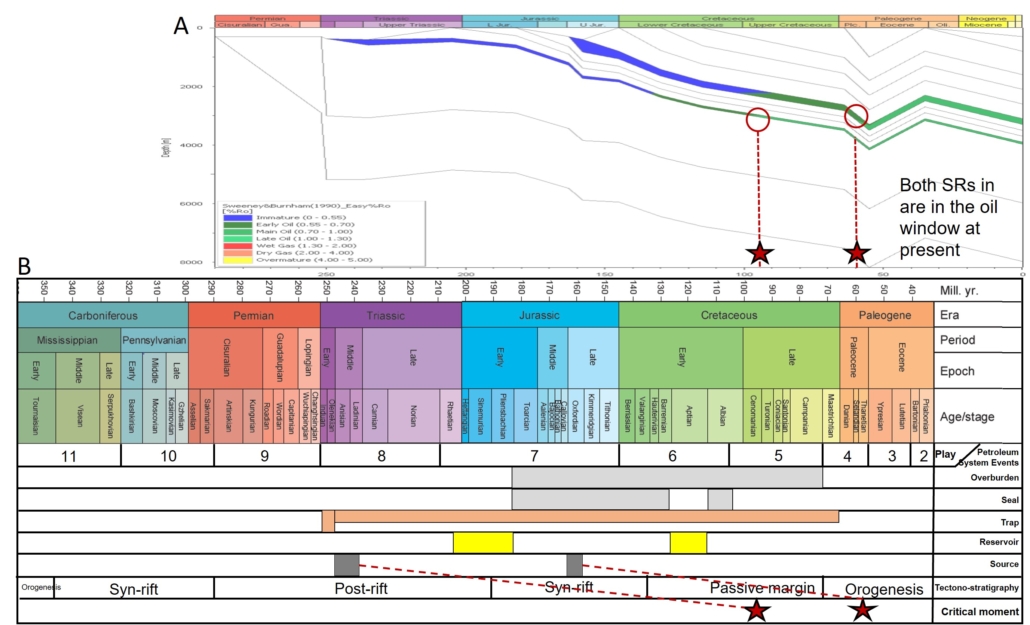
Figure 20 – A: Subsidence curve with VitRef maturity (EasyR0) overlay. Diagram is showing that the Middle Triassic source rock enters the main oil window in early Late Cretaceous and the Late Jurassic source rock enters the main oil window in Late Paleocene.
B: Petroleum System Event Chart showing timing of deposition of play elements and critical moments. Critical moments are when the two source rocks of Middle Triassic and Late Jurassic age enter the main oil window and migration of hydrocarbons starts.
Assessment Process
The assessment process for the resource evaluation of AU7 is summarised in the “Yet-To-Find Triangle” shown in Figure 21. Basin evaluation is the basic activity describing the structural and stratigraphic framework leading into construction of Gross Depositional Environment (GDE) maps and identification of source rocks, reservoirs and regional seals that form the basis for definition of the plays.
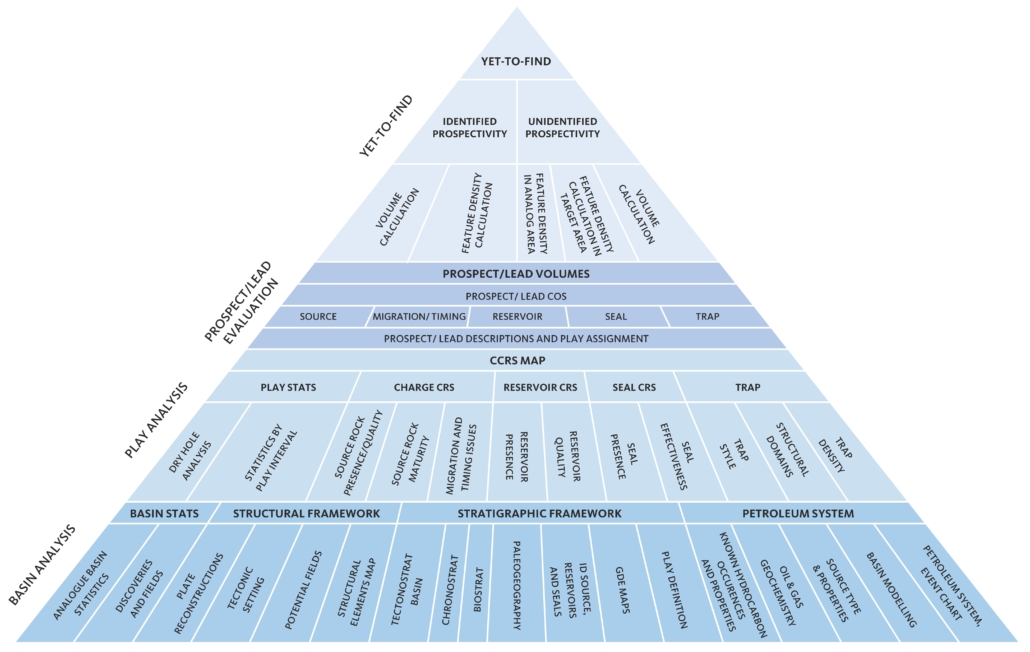
Figure 21 – Resource evaluation workflow used in the Greenland Resource Assessment.
The results from the Basin Evaluation feed into the Play Analysis. In this analysis, numeric Common Risk Segment (CRS) and Composite Common Risk Segment (CCRS) maps have been constructed based on all available geological and geophysical data.
For the Franklinian Basin, the standard risking schemes used in Assessment Units 1–5 have been used. However, for the Lincoln Sea Basin risking has not been carried out on individual plays but only on general, overall leve lassessing the following play risk elements have been assessed (see presentation for details):
• Basin presence: Validity of Lincoln Sea Basin model including presence of Carboniferous salt section.
• Reservoir presence: Presence of at least one effective reservoir unit in the subsurface with a possible connection to source.
• Reservoir effectiveness: Anticipated burial of possible reservoir unit corrected for uplift and possibility of degrading intrusions.
• Top seal effectiveness: Overburden thickness, modified in areas with large amount of uplift.
• Trap presence: Possibility to acquire high-quality data.
• Charge: Data from analogue basins (possible presence of Triassic and Jurassic source).
Gas risk has not been assessed and all volumes are in MMBOE. For analysis on volumetrics of individual phases, more detailed analysis and mapping must be carried out.
Resource Assessment
The resource assessment provides an estimate of the play-based Yet-to-Find potential of conventional hydrocarbons. The Franklinian Basin has not been assessed since total risks for the two defined play are too high to produce a meaningful estimate (Fig. 22).
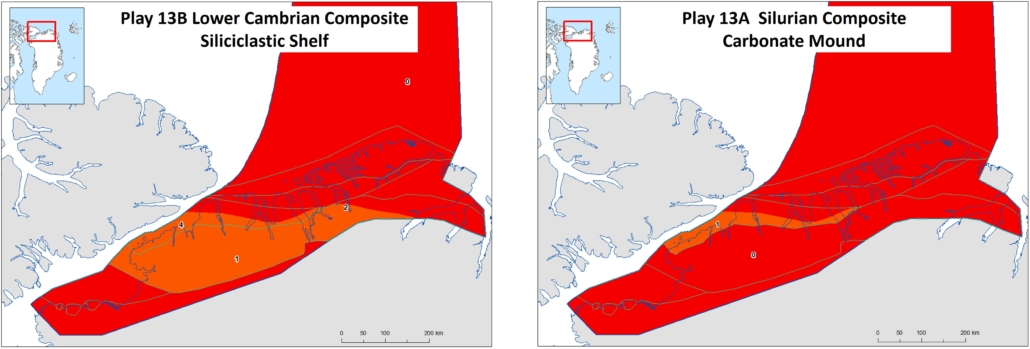
Figure 22 – Total Risk maps for the two analysed plays of the Franklinian Basin. Both show very high risk, and no Yet-to-Find analysis has therefore been carried out.
As mentioned above, the assessment of the Lincoln Sea Basin is only carried out on a general, overall level and no known prospectivity can be included.
The Yet-to-Find analysis is based on a feature (lead) density calculation approach which is based on analogue data from the Sverdrup Basin (Fig 23).
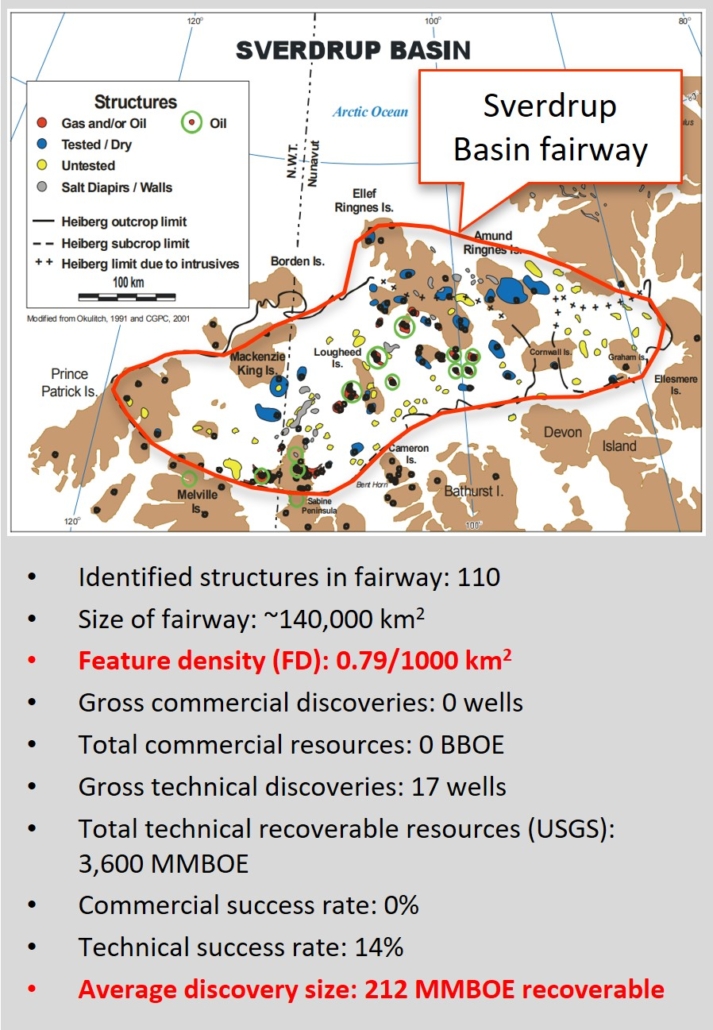
Figure 23 – Exploration data for the Sverdrup Basin fairway. Mapped features from Meneley (2008), resource data from Tennyson & Pitman (2008).
The Mean Risked Recoverable of the Lincoln Sea Basin is:
Sum of: [SegmentArea*FD*MFV*AU6TotalRiskOverall(per area)]
The Sverdrup Basin fairway (~140,000 km2) is regarded to be almost fully explored by now. 110 structures have been identified in the fairway (Meneley 2008) corresponding to a feature density (FD) of 0.79/1000 km2. The total amount of technical resources amounts to 3.6 BBOE from 17 wells (Tennyson & Pitman 2008) corresponding to an average unrisked discovery size (mean feature volume – MFV) of 212 MMBOE per well.
The total risked Yet-to-Find of the Greenland part of the Lincoln Sea Basin is thus 800 MMBOE including the thinner rim of the basin (Fig. 24).
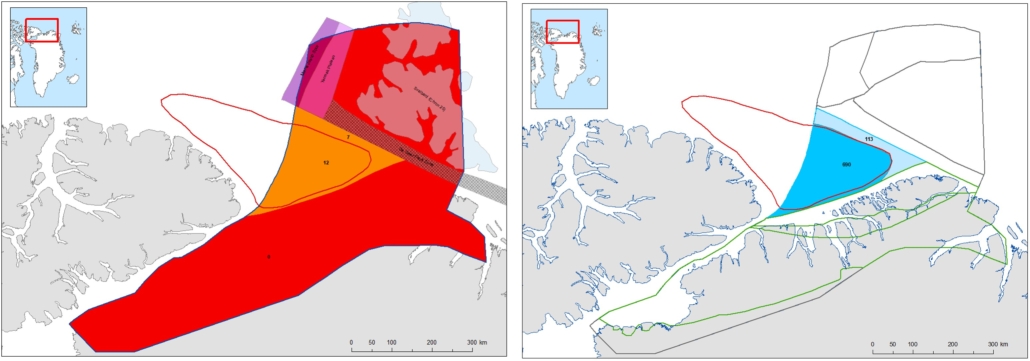
Figure 24 – Total risk map and risked recoverables in MMBOE for the Greenland part of the Lincoln Sea Basin.
Deliverables
The results from this study including a Player® ArcGIS project and an extensive slide pack are available for the petroleum exploration industry by contacting Thomas Varming at NUNAOIL.
References
Abay, T. B., Karlsen, D. A., Lerch, B., Olaussen, S., Pedersen, J. H. & Backer-Owe, K. 2017: Migrated petroleum in outcropping Mesozoic sedimentary rocks in Spitsbergen: Organic geochemical characterization and implications for regional exploration. Journal of Petroleum Geology 40(1), 5-36. https://doi.org/10.1144/petgeo2017-035
Alsen, P., McRoberts, C., Svennevig, K., Bojesen-Koefoed, J. A., Hovikoski, J. & Piasecki, S. 2017: The Isrand Formation: a Middle Triassic Daonella-bearing, black shale unit in Kilen, North Greenland (with a note on the Triassic in Amdrup Land). Newsletters on Stratigraphy 50, 31–46. https://doi.org/10.1127/nos/2016/0341
Berglar, K., et al. 2022: Petroleum potential of the northern Barents Sea and the Northeast Greenland margin including aspects of oil spill related environmental risks of petroleum production in the Arctic. – Final report of project PANORAMA. 327 pp pp. https://doi.org/10.25928/bgr_panorama.
Bjerager, M., Alsen, P., Bojesen-Koefoed, J. A., Fyhn, M. B. W., Hovikoski, J., Keulen, N., Lindström, S., Therkelsen, J. & Thomsen, T. B. 2023: Triassic in the northernmost Atlantic—Linking North Greenland and the southwestern Barents Sea. Terra Nova, 10. https://doi.org/10.1111/ter.12649
Christiansen, F. G., (ed.) 1989: Petroleum geology of North Greenland. Bulletin Grønlands Geologiske Undersøgelse 158, 104 pp. https://geusjournals.org/index.php/bullggu/issue/view/610/53
Christiansen, F. G. & Bojesen-Koefoed, J. A. 2021: Inventory of onshore petroleum seeps and stains in Greenland: a web-based GIS model. GEUS Bulletin 47, 1-20. https://doi.org/10.34194/geusb.v47.6519
Christiansen, F. G., Nøhr-Hansen, H. & Nykjær, O. 1987: The Cambrian Henson Gletscher Formation: a mature to post-rnature hydrocarbon source rock sequence from North Greenland. Rapport Grønlands Geologiske Undersøgelse 133, 141-157. https://doi.org/10.34194/rapggu.v133.7984
Dalhoff, F. & Stemmerik, L. 2000: Depositional history of the fluvial Lower Carboniferous Sortebakker Formation, Wandel Sea Basin, eastern North Greenland. Geology of Greenland Survey Bulletin 187, 65-77. https://doi.org/10.34194/ggub.v187.5195
Dallmann, W. K., Blomeier, D., Elvevold, S., Mørk, A., Olaussen, S., Grundvåg, S.-A., Bond, D. & Hormes, A. 2015: Chapter 6. Historical geology. In: Dallmann, W. K. (ed.) Geoscience Atlas of Svalbard. Norsk Polarinstitutt Rapportserie 148, 89-132. http://hdl.handle.net/11250/2580810
Dypvik, H., Håkansson, E. & Heinberg, C. 2002: Jurassic and Cretaceous palaeogeography and stratigraphic comparisons in the North Greenland–Svalbard region. Polar Research 21(1), 91–108. https://doi.org/10.1111/j.1751-8369.2002.tb00069.x
Embry, A. & Beauchamp, B. 2008: Chapter 13. Sverdrup Basin. In: Miall, A. D. (ed.) Sedimentary basins of the United States and Canada. Sedimentary basins of the World 5, 451–471. https://doi.org/10.1016/S1874-5997(08)00013-0
Embry, A. & Beauchamp, B. 2019: Chapter 14 -Sverdrup Basin. In: Miall, A. D. (ed.) The sedimentary basins of the United States and Canada, 559-592. Elseveir. https://doi.org/10.1016/B978-0-444-63895-3.00014-0.
Forsyth, D. A., Argyle, M., Okulitch, A. & Trettin, H. P. 1994: New seismic, magnetic, and gravity constraints on the crustal structure on the Lincoln Sea continent–ocean transition. Canadian Journal of Earth Science 31, 905–918. https://doi.org/10.1139/e94-082
Funck, T., Dehler, S. A., Jackson, H. R., Salisbury, M. H. & Reid, I. D. 2006: A refraction seismic image of the sediments in Kennedy Channel, northern Nares Strait. Polarforschung 74(1-3), 41–50. https://doi.org/10.2312/polarforschung.74.1-3.41
Galloway, B. J., Dewing, K. & Beauchamp, B. 2018: Upper Paleozoic hydrocarbon systems in the Sverdrup Basin, Canadian Arctic Islands. Marine and Petroleum Geology 92, 809-821. https://doi.org/10.1016/j.marpetgeo.2017.12.013
Gautier, D. & Moore, T. 2008 Chapter A: Introduction to the 2008 Circum-Arctic Resource Appraisal (CARA) In: Moore, T. & Gautier, D. (eds): The 2008 Circum-Arctic Resource Appraisal. U.S. Geological Survey Professional Paper 1824, 9 pp. https://doi.org/10.3133/pp1824A.
Goodarzi, F., Gentzis, T., Embry, A. F., Osadtez, K. G., Skibo, D. N. & Stewart, K. R. 1992: Evaluation of maturity and source rock potential in the Lougheed Island area of the central Sverdrup Basin, Arctic Canada. In: Vorren, T. O., et al. (eds): Arctic geology and petroleum potential. NPF Special Publication 2, 147–157. https://doi.org/10.1016/B978-0-444-88943-0.50014-9.
Hadlari, T., Midwinter, D., Galloway, J. M., Dewing, K. & Durbano, A. M. 2016: Mesozoic rift to post-rift tectonostratigraphy of the Sverdrup Basin, Canadian Arctic. Marine and Petroleum Geology 76, 148-158. http://dx.doi.org/10.1016/j.marpetgeo.2016.05.008
Herrle, J. O., Schröder-Adams, C. J., Davis, W., Pugh, A. T., Galloway, J. M. & Fath, J. 2015: Mid-Cretaceous High Arctic stratigraphy, climate, and Oceanic Anoxic Events. Geology 43, 403–406. https://doi.org/10.1130/G36439.1
Higgins, A. K., Ineson, J. R., Peel, J. S., Surlyk, F. & Sønderholm, M. 1991: Lower Palaeozoic Franklinian Basin of North Greenland. In: Peel, J. S. & Sønderholm, M. (eds): Sedimentary basins of North Greenland. Bulletin Grønlands Geologiske Undersøgelse 160, 71–140. https://doi.org/10.34194/bullggu.v160.6714
Hopper, J. R. & Ineson, J. R. 2021: Franklinian composite tectono-sedimentary element, North Greenland In: Drachev, S. S., et al. (eds): Sedimentary successions of the Arctic Region. Geological Society Memoir 57, 13 pp. http://mr.crossref.org/iPage?doi=10.1144%2FM57-2020-6
Håkansson, E., Heinberg, C. & Stemmerik, L. 1991: Mesozoic and Cenozoic history of the Wandel Sea Basin area, North Greenland. In: Peel, J. S. & Sønderholm, M. (eds): Sedimentary basins of North Greenland. Bulletin Grønlands Geologiske Undersøgelse 160, 153–164. https://doi.org/10.34194/bullggu.v160.6716
Ineson, J. R. & Peel, J. S. 1997: Cambrian shelf stratigraphy of North Greenland. Geology of Greenland Survey Bulletin 173, 120 pp. https://doi.org/10.34194/ggub.v173.5024
Jackson, H. R., Dahl-Jensen, T. & group, L. w. 2010: Sedimentary and crustal structure from the Ellesmere Island and Greenland continental shelves onto the Lomonosov Ridge, Arctic Ocean. Geophysical Journal International 182, 11-35. https://10.1111/j.1365-246X.2010.04604.x
Kovacs, L. C. 1982: Motion along Nares Strait recorded in the Lincoln Sea: aeromagnetic evidence. Meddelelser om Grønland, Geoscience 8, 275–290.
Kristoffersen, Y., Hall, J. K. & Nilsen, E. H. 2021: Morris Jesup Spur and Rise north of Greenland – exploring present seabed features, the history of sediment deposition, volcanism and tectonic deformation at a Late Cretaceous/early Cenozoic triple junction in the Arctic Ocean. Norwegian Journal of Geology 101, 202104. https://dx.doi.org/10.17850/njg101-1-4
Lebedeva‐Ivanova, N., Gaina, C., Minakov, A. & Kashubin, S. 2019: ArcCRUST: Arctic Crustal Thickness From 3‐D Gravity Inversion. Geochemistry, Geophysics, Geosystems 20, 3225-3247. https://doi.org/10.1029/2018GC008098
Leith, T. H., et al., (eds) 1993: Mesozoic hydrocarbon source-rocks of the Arctic region. Norwegian Petroleum Society Special Publications 2, 1-25 pp. https://doi.org/10.1016/b978-0-444-88943-0.50006-x.
Lerch, B. 2016: Petroleum systems of the Barents Sea, 95 pp. Unpublished Ph.D. Thesis, University of Oslo.
Lerch, B., Karlsen, D. A., Matapour, Z., Seland, R. & Backer-Owe, K. 2016: Organic geochemistry of Barents Sea Petroleum: Thermal maturity and alteration and mixing processes in oils and condensates. Journal of Petroleum Geology 39(2), 125-148. https://doi.org/10.1111/jpg.12637
Lister, C. J., Atkinson, E. A., Dewing, K. E., King, H. M., Kung, L. U. & Hadlari, T. 2022: High Arctic basins petroleum potential, northern Canada. Geological Survey of Canada, Open File 8897, 88 pp. https://doi.org/10.4095/330203
Lundschien, B. A., Høy, T. & Mørk, A. 2014: Triassic hydrocarbon potential in the Northern Barents Sea; integrating Svalbard and stratigraphic core data. Norwegian Petroleum Directorate Bulletin 11, 3-20.
Matapour, Z. & Karlsen, D. A. 2018: Ages of Norwegian oils and bitumen based on age-specific biomarkers. Petroleum Geoscience 24, 92–101. https://doi.org/10.1144/petgeo2016-119
McMillan, N. J. 1982: Nares Strait and the petroleum explorer. Meddelelser om Grønland, Geoscience 8, 355–361.
Meneley, R. 2008: The significance of oil the Sverdrup Basin. CSPG/CSEG/CWLS GeoConvention, Calgary, Alberta, Canada, May 12–15 2008. https://www.searchanddiscovery.com/abstracts/pdf/2013/90170cspg/abstracts/ndx_menel.pdf
Mørk, A., et al. 1999: Mesozoic lithostratigraphy. In: Dallmann, W. (ed.) Lithostratigraphic lexicon of Svalbard: Review and recommendations for nomenclature use. Upper Palaeozoic to Quaternary Bedrock, 127–214. Tromsø: Norsk Polarinstitut.
Nøttvedt, A., Cecchi, M., Gjelberg, J. G., Kristensen, S. E., Lønøy, A., Rasmussen, A., Rasmussen, E., Skott, P. H. & van Veen, P. M. 1993: Svalbard-Barents Sea correlation: a short review. In: Vorren, T. O., et al. (eds): Arctic geology and petroleum potential. Norwegian Petroleum Society Special Publications 2 https://doi.org/10.1016/B978-0-444-88943-0.50027-7
Oakey, G. & Chalmers, J. 2001: Constraints on the kinematic model of Tertiary motion of Greenland. GAC/MAC Annual Meeting, St. John’s, Newfoundland, Canada.
Ohm, S. E., Karlsen, D. A. & Austin, T. J. F. 2009: Geochemically driven exploration models in uplifted areas: Examples from the Norwegian Barents Sea. AAPG Bulletin 92(9), 1191–1223. https://doi.org/10.1306/06180808028
Olaussen, S., et al. 2022: Svalbard Composite Tectono-Sedimentary Element, Barents Sea. In: Drachev, S. S., Brekke, H., Henriksen, E. and Moore, T. (ed.) Sedimentary Successions of the Arctic Region and their Hydrocarbon Prospectivity. Geological Society, London, Memoirs. https://doi.org/10.1144/M57-2021-36.
Pedersen, J. H., Karlsen, D. A., Brunstad, H. & Lie, J. E. 2005: Oil and gas of the Norwegian Barents Sea. AAPG Annual Meeting. Calgary, Alberta, June 16–19, 2005 https://www.yumpu.com/en/document/read/31843831/source-rocks-of-the-norwegian-barents-sea-i-oil-and-gas-geology
Pepper, A. S. & Corvi, P. J. 1995: Simple kinetic models of petroleum fomation. Part I: Oil and gas generation from kerogen. Marine and Petroleum Geology 12(3), 291–319. https://doi.org/10.1016/0264-8172(95)98381-E
Stemmerik, L., Bojesen-Koefoed, J. A. & Sønderholm, M. 1997: Palaeo-oil field in a Silurian carbonate buildup, Wulff Land, North Greenland: project ‘Resources of the sedimentary basins of North and East Greenland. Geology of Greenland Survey Bulletin 176, 24–28. https://doi.org/10.34194/ggub.v176.5056
Stemmerik, L. & Håkansson, E. 1991: Carboniferous and Permian history of the Wandel Sea Basin, North Greenland. In: Peel, J. S. & Sønderholm, M. (eds): Sedimentary basins of North Greenland. Bulletin Grønlands Geologiske Undersøgelse 160, 141-151. https://doi.org/10.34194/bullggu.v160.6715
Stemmerik, L. & Worsley, D. 2005: 30 years on – Arctic Upper Palaeozoic stratigraphy, depositional evolution and hydrocarbon prospectivity. Norwegian Journal of Geology 85, 151-168.
Surlyk, F. 1991: Tectonostratigraphy of North Greenland. In: Peel, J. S. & Sønderholm, M. (eds): Sedimentary basins of North Greenland. Bulletin Grønlands Geologiske Undersøgele 160, 25–47. https://doi.org/10.34194/bullggu.v160.6712
Sørensen, K., Gautier, D., Pitman, J., Jackson, J. & Dahl-Jensen, T. 2011: Geology and petroleum potential of the Lincoln Sea Basin, offshore North Greenland. In: Spencer, A. M., et al. (eds): Arctic Petroleum Geology. Geological Society Memoir 35, 673–684. https://doi.org/10.1144/M35.44.
Tennyson, M. & Pitman, J. 2008: Chapter H: Geology and assessment of undiscovered oil and gas resources of the Franklinian Shelf Province, Arctic Canada and North Greenland. In: Moore, T. E. & Gautier, D. L. (eds): The 2008 Circum-Arctic Resource Appraisal. U.S. Geological Survey Professional Paper 1824, 19 pp. . https://doi.org/10.3133/pp1824H.
van Koeverden, J. H., Karlsen, D. A. & Backer-Owe, K. 2011: Carboniferous non-marine source rocks from Spitsbergen and Bjørnøya: Comarison with Western Arctic. Journal of Petroleum Geology 34(1), 53-66.
van Koeverden, J. H., Karlsen, D. A., Schwark, L., Chpitsglouz, A. & Backer-Owe, K. 2010: Oil-prone Lower Carboniferous coals in the Norwegian Barents Sea: Implications for a Palaeozoic petroleum system. Journal of Petroleum Geology 33(2), 155-181. https://doi.org/10.1111/j.1747-5457.2010.00471.x
Wesenlund, F., Engelschiøn, V. S., Thießen, O. & Pedersen, J. H. 2022: Multi-elemental chemostratigraphy of Triassic mudstones in eastern Svalbard: Implications for source rock formation in front of the World’s largest delta plain. The Depositional record 8(2), 718-753. https://doi.org/10.1002/dep2.182
Wesenlund, F., Grundvåg, S. A., Engelschiøn, V. S., Thießen, O. & Pedersen, J. H. 2021: Linking facies variations, organic carbon richness and bulk bitumen content – A case study of the organic-rich Middle Triassic shales from eastern Svalbard. Marine and Petroleum Geology 132, 105168. https://doi.org/10.1016/j.marpetgeo.2021.105168