Greenland Resource Assessment,
Central East Greenland
(Assessment Unit 5)
Executive Summary
A play-based resource assessment of conventional hydrocarbons has been performed for the onshore and offshore areas of Central East Greenland (Assessment Unit 5, AU5) which is part of the major rift complex formed during the opening of the North Atlantic. Three play levels have been fully assessed in the potentially prospective area covering 126,956 km2 – Permian, Jurassic and Early Cretaceous. All other plays (Devonian, Carboniferous, Triassic, Late Cretaceous, latest Cretaceous – Paleocene and Eocene) have been fully analysed but are excluded from the Yet-to-Find assessment since prospectivity of these plays is very low due to various reasons such as very deep burial, lack of regional source, lack of regional seal or severe charge issues.
Since onshore seismic data are of very low quality and because offshore data are missing, analogue feature densities have been obtained from well-explored areas in Assessment Area 4 (North-East Greenland) which show a similar structural development as the areas in question in AU5.
The known prospectivity includes only 4 leads from mapped at Carboniferous and Permian levels onshore in Jameson Land with mean risked recoverable volumes of ~27 MMBOE. The undiscovered resources have an estimated mean of ~2,300 MMBOE risked recoverable conventional hydrocarbons. Thus, the total prospectivity has mean risked recoverable volumes of ~2350 MMBOE resulting in an average area yield of ~3 MB/1000 km2 (risked). However, the northern onshore subareas show average yields of ~85 MB/1000 km2 (risked).
The geologic probabilities for reservoir quality, reservoir effectiveness, seal effectiveness, trap presence and charge for AU5 were determined based on careful play mapping constructing a series of numeric CRS (Common Risk Segment) maps and CCRS (Composite Common Risk Segment) maps of the evaluated plays in the assessment area. In this manner, the probabilities are consistently applied throughout the area for both the known and unknown prospectivity.
The assessment results presented are based on all available data collected and interpreted by the industry, GEUS, NUNAOIL and the Government of Greenland and reflect the state of geologic knowledge of the offshore and onshore areas of North-East Greenland at the time of study. Any future drilling and evaluation of the petroleum systems and plays based on new data within this area will add to the geologic knowledge and will therefore lead to a refinement of these assessment results.
The assessment was carried out using the Player® GIS software provided by GIS-pax. The methodology and results of the assessment have been quality controlled by the Norwegian Petroleum Directorate and GIS-pax.
The results of this study are reported in an extensive ArcGIS project supported by a comprehensive slide package which includes 10 presentations, reflecting the workflow of the project, including:
- Introduction
- Database
- Structural Framework
- Stratigraphic Framework
- Seismic-Stratigraphic Framework
- Petroleum Systems
- Play Analysis
- Lead Overview
- Input Parameters
- Yet-to-Find Analysis
The Player and ArcGIS projects together with the slide pack are available for the petroleum exploration industry at no cost and can be downloaded from the project website, www.greenland-resource-assessment.gl.
Introduction
The AU5 Resource Assessment (Central East Greenland) is the fifth of seven assessments of the “Whole-of-Greenland Petroleum Resource Assessment Project” covering the entire Greenland continental shelf and adjacent onshore basins (Fig. 1). The aim of the project is to provide an estimate of a quantitative, play-based Yet-to-Find potential of conventional hydrocarbons on the Greenland continental shelf. The project was originally initiated to help the Greenland Authorities and politicians in strategic decision making and in planning of future natural resource administration. In addition, it should identify potential needs for further geological and geophysical investigations.
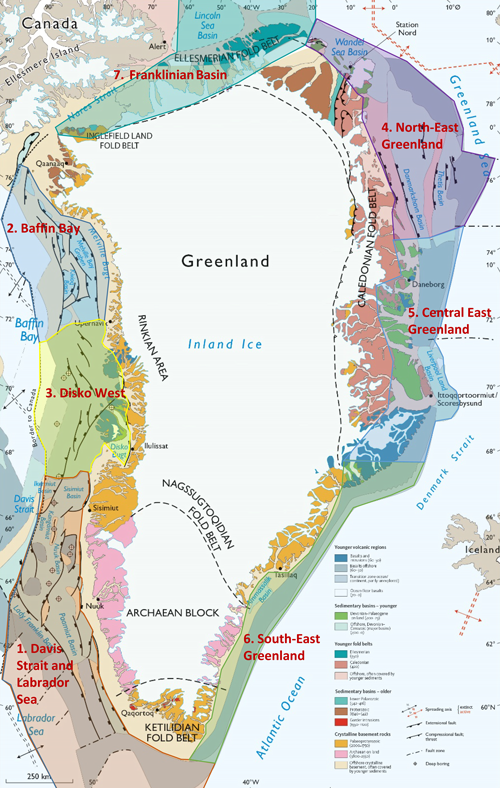
Figure 1 – Geological map of Greenland with the seven assessment units indicated.
The project is mainly sponsored by NUNAOIL and is carried out as a collaboration between GEUS, NUNAOIL and the Government of Greenland hosted by GEUS. The work is based on all available existing data provided by the industry, GEUS, NUNAOIL and the Greenland Government. Furthermore, it includes a regional basin modelling study carried out in-house at GEUS. The results are reported as the work on the individual assessment units are finalized.
A hydrocarbon resource assessment of the Greenland continental shelf north of the Arctic Circle has previously been performed as part of the U.S. Geological Survey (USGS) Circum-Arctic Resource Program (Gautier 2017). In this study resources in the order of 700 MMBOE risked recoverable were reported from the offshore Central East Greenland Rift Basin (Liverpool Land Basin) and the onshore basins were not assessed. Furthermore, the USGS assessment used a probabilistic approach heavily relying on analogue modelling comparing the Arctic assessment units with similar but geologically better understood and better calibrated petroleum provinces elsewhere in the world. This study therefore only provided a broad overview of the possible resource potential within the assessment units of the study.
Database
The database for AU5 includes an open, onshore and offshore 2D seismic grid (~31,000 km) and gravity surveys of fairly old vintage (most are pre 2000), one offshore and 59 onshore stratigraphic boreholes drilled providing information on Permian to Neogene plays (Fig. 2). Numerous scientific publications and industry reports with data are available. Excellent outcrops exist in the onshore area. The extensive amount of data from the mid-Norwegian Shelf has also been used as analogues where appropriate.
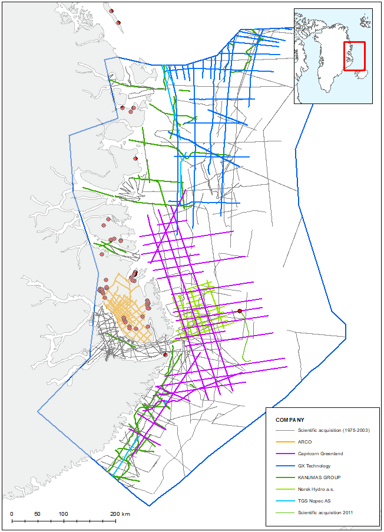
Figure 2 – Seismic database of AU5. Red dots are stratigraphic boreholes.
Exploration History
Exploration efforts in East Greenland have been carried out intermittently since 1985.
1. Arco Greenland held a licence onshore Jameson Land during 1985–1990. An open 2D seismic grid totalling 1800 km was acquired (Fig.2).
2. In 1989, the KANUMAS-group (Kalaallit Nunaat Marine Seismic Project) – including BP, Chevron, Exxon, JNOC, Shell, Statoil and NUNAOIL – was awarded a prospecting licence with special preferential rights in Baffin Bay and offshore North-East and East Greenland. Roughly 2750 km of 2D seismic data were acquired offshore Central East Greenland during 1991–1995 as part of the KANUMAS project (Fig. 2).
3. In 2006–2007 Norsk Hydro acquired around 1290 km additional seismic data (Fig. 2).
4. Between 2008 and 2011, TGS, Capricorn Greenland and Ion acquired more than 8000 km 2D seismic data ahead of the announced opening up for subsurface exploration licensing in 2012 (Fig. 2).
5. In 2015 a small company, Greenland Gas and Oil, acquired two licences onshore Jameson Land without seismic data being acquired.
Additional scientific 2D seismic data have been acquired by the Geological Survey of Greenland (c. 7100 km around 1980), the Alfred Wegener Institute (c. 4050 km during 1994–2003), the Bundesanstalt für Geologie und Rohstoffe (c. 4060 km during 1975–1988) and the University of Tromsø (c. 100 km in 2011; Fig. 2).
Seismic-Stratigraphic Interpretation
The regional seismic stratigraphic interpretation is based on a GEUS and NUNAOIL in-house interpretation. In the offshore part of AU5, two Cenozoic super-regional seismic stratigraphic key horizons (Intra Middle Miocene and Top Basalt) in addition to the seabed have been picked within AU4. These horizons were correlated with roughly coeval stratigraphic intervals cored in shallow stratigraphic boreholes and the ODP 913 borehole located in AU 4 farther north. In addition, the Top Basalt is dated to the early Eocene onshore, although some diachroneity exists across the area. The basalt forms an acoustic basement and any sediments older than the Eocene cannot be resolved seismically. A thick sub-basaltic section is anticipated in areas forming the offshore continuation of the Devonian–Paleocene section exposed onshore and occurring immediately north of the basalts within AU 4. In addition to the three regional surfaces, sub-regional surfaces have been picked in order to further constrain the geological evolution that includes an Eocene-Oligocene extensional phase and later episodes of uplift and erosion presumably commencing around the latest Oligocene – both affecting the inner margin and onshore areas (Fig. 3).
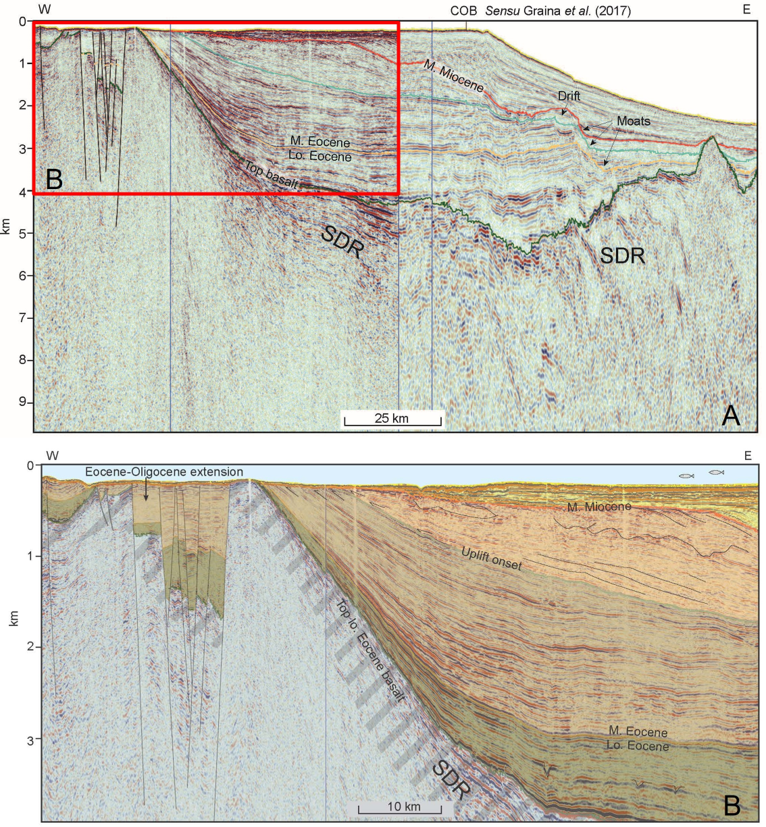
Figure 3 – A: Regional seismic transect from offshore Wollaston Forland and into the oceanic realm of AU5 illustrating the geology and mapped seismic stratigraphy. B: Zoom-in with colorized stratigraphy on the inner part of the margin documenting an Eocene extensional phase and the response of uplift, erosion and associated changes in offshore deposition. SDR: Seaward dipping reflectors.
The seismic stratigraphy within the onshore Jameson Land Basin has been subdivided into 11 units correlated with outcropping stratigraphy and shallow boreholes (Fig. 4). The regional seismic stratigraphic key horizons that have been mapped are: A: Surface; B: Intra Early Cretaceous (Top Hesteelv Fm); C: Base Late Jurassic (Top Fossilbjerget Fm); D: intra Middle Jurassic (Top Neill Klinter Gp); E: Intra Early Jurassic (Top Kap Stewart Gp); F: Intra Triassic (Top Fleming Fjord Gp); G: Intra Triassic (Top Gipsdalen Fm?); H: Intra Triassic (Top Pingo Dal Gp?); I: Intra Triassic (Top Wordie Creek Gp); J: Near base Triassic (Base Wordie Creek Gp?); K: Near base Permian, and L: Near base Carboniferous.
The Jameson Land Basin is heavily intruded by basalt sills producing an irregular strong reflectivity which overprints and dampens the stratigraphic signal complicating the structural and stratigraphic interpretation in the basin. The stratigraphic mapping is therefore associated with considerable uncertainty.
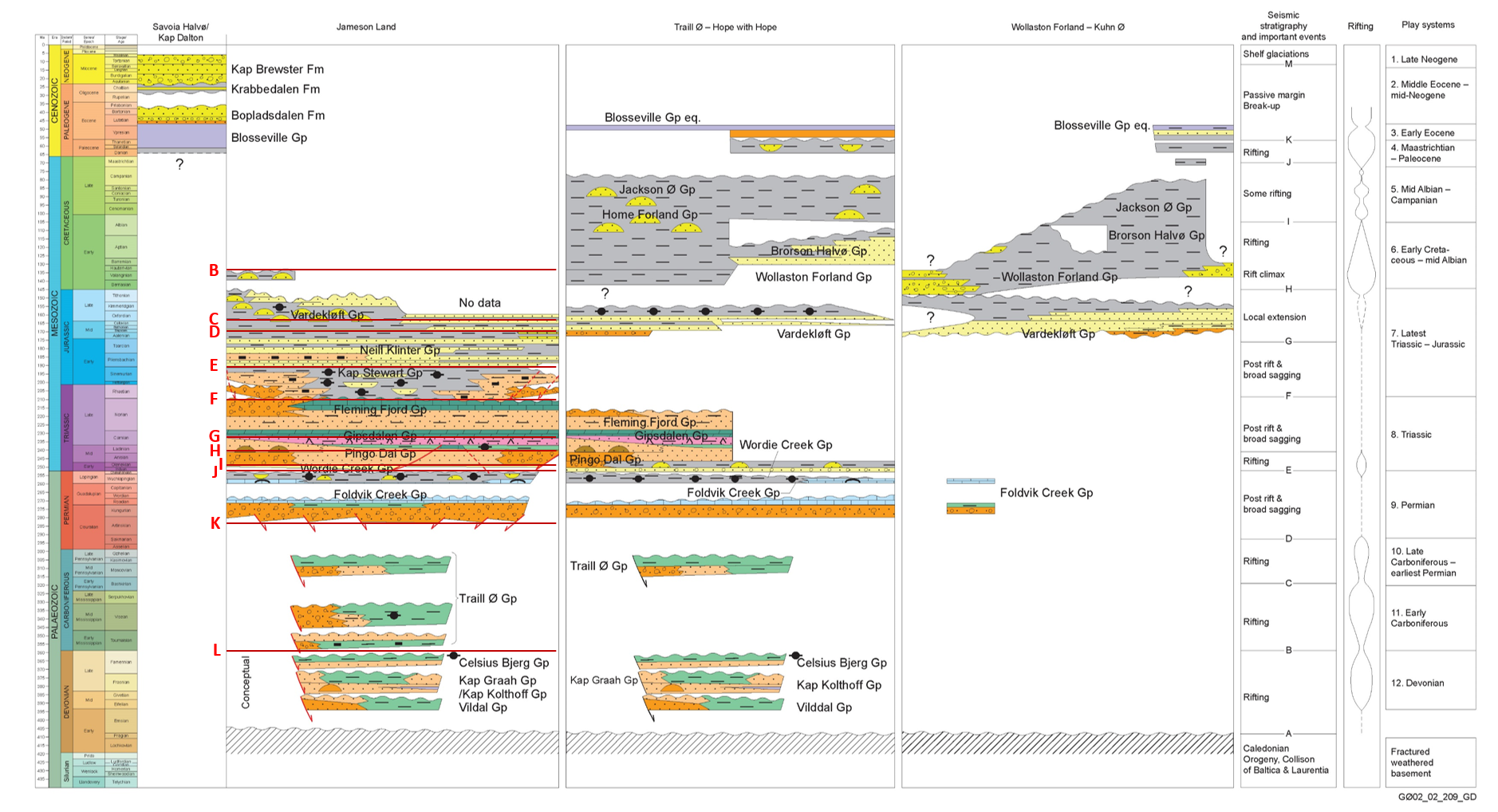
Figure 4 – Stratigraphy interpreted in the onshore part of AU5. Seismic stratigraphy within the Jameson Land Basin indicated with red lines.
Tectonostratigraphic Evolution
Assessment Unit AU5 (central East Greenland) is part of the NE Atlantic rift system and embraces the East Greenland Rift System of Surlyk (1978) and the Central East Greenland Composite Tectono-Sedimentary Element of Fyhn et al. (in press). It is bounded to the west by the Post-Devonian Main Fault, to the east by the National Sector Boundary, to the south by the intersection of the possible extension of the onshore rift basin and the COB along Blosseville Kyst (Fig. 5). The northern boundary is roughly placed along the southern extension of the Danmarkshavn Basin along the boundary to the North-East Greenland Composite Tectono-Sedimentary Element (Fyhn & Hopper, in press). In the offshore areas, large parts of AU5 is floored by oceanic crust or covered by Palaeogene basalts.
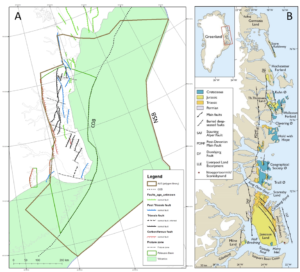
Figure 5 – A: Outline of Assessment Unit AU5 showing main fault systems. COB: Continent–Ocean Boundary; NSB: National Sector Boundary. B: Simplified geological map of the East Greenland Rift Basin showing the main faults being active during the Jurassic, the main areas with outcrops of uppermost Jurassic – lowermost Cretaceous formations. From Surlyk et al. (2021).
In most of the onshore areas the outlines of the rift basins are well constrained because of the nature of the world-class outcrops. However, in the southern part of AU5, south of Scoresby Sund, basin outlines are not known due to the overlying thick succession of Palaeogene volcanic rocks and the lack of good seismic data. The rift system is anticipated to extend into the offshore areas but is covered by at hick succession of Palaeogene basalt that prevents imaging of the sub-volcanic sedimentary successions.
The tectonic evolution of the East Greenland Rift System is complex involving the Devonian collapse of the Caledonian Orogen followed by multiple phases of rifting, related to the separation and eventual break-up of Greenland and Eurasia as well as intervening phases of tectonic quiescence (Fig. 4; e.g. Surlyk, 1978; Clemmensen, 1980a, b; Olsen, 1993; Olsen & Larsen, 1993; Vigran et al., 1999; Stemmerik, 2001; Seidler et al., 2004; Surlyk et al.. 2021; Hovikoski et al., 2012, 2021; Ineson et al., 2020; Bjerager et al., 2020; Fyhn & Hopper, in press). Furthermore, the region is strongly affected by the North Atlantic Mantle Plume, resulting in Paleocene and early Eocene intrusive activity and flood magmatism. Intrusions in the Jameson Land Basin strongly hamper seismic imaging and restrict the level of detail to which structural and stratigraphic interpretation is possible with the existing seismic dataset. Similarly, in the offshore areas pre-volcanic imaging is hampered by the widespread basalt cover.
In AU5 the East Greenland Rift system comprises several basins and sub-basins formed during a succession of rift phases during the Mesozoic. Sedimentation occurred in a system of grabens and half-grabens that are bounded by transfer fault zones in the fjord areas (Fig. 5A). These rift basins and sub-basins are characterized by having their own depositional evolution, whereas during post-rift phases they were connected and had a common depositional history.
Twelve major tectonostratigraphic phases have been recognized in central East Greenland: (12) Devonian, (11) Early Carboniferous, (10) Late Carboniferous – earliest Permian, (9) Permian, (8) Triassic, (7) Latest Triassic – Jurassic, (6) Earliest Cretaceous – mid-Albian, (5) Mid Albian – Campanian, (4) Maastrichtian-Paleocene, (3) Early Eocene, Middle Eocene – mid-Neogene, (1) Late Neogene (Fig. 4).
The sediments of the East Greenland Rift System in AU5 are underlain and flanked by Precambrian gneisses and Palaeozoic felsic intrusions and Proterozoic and early Palaeozoic sediments.
The East Greenland Rift Basin was initiated in the Middle Devonian (Larsen & Bengaard, 1991). Several tectonic models have been proposed to explain the initiation and development of the basin, but most models relate basin formation to an E-W extensional collapse of an overthickened Caledonian welt (Larsen & Bengaard, 1991). The extension was suggested to have been accommodated along two major NNE–SSW–trending east-dipping normal faults that transect the crystalline basement and Caledonian fold structures west of the present-day outcrop of the Devonian basin.
During deposition the basin underwent a series of deformation events that continued into the Early Carboniferous (Larsen & Bengaard, 1991; Guarnieri et al., 2021). These deformation events are suggested to have been caused by left-lateral strike-slip movements along the eastern basin margin (Larsen & Bengaard, 1991). During the basin evolution more than 8 km of continental clastic deposits accumulated (e.g. Olsen & Larsen, 1993). Rifting continued into the Carboniferous along N–S trending faults, resulting in mainly westward tilted blocks creating N–S trending half grabens. The syn-rift deposits are up to several km in thickness and include alluvial, fluvial and lacustrine deltaic sandstones and lacustrine mudstones in places deposited locally under anoxic conditions (Fig. 4; Vigran et al., 1999).
Carboniferous rifting was followed by broad post-rift sagging of the rift system (Stemmerik, 2001). Syn-rift deposits are separated from the Permian post-rift succession by an angular unconformity (Permian peneplain), in places associated with valley incision, overlain by low-relief braid plain sandstones and conglomerates of possible Early–Middle Permian in age. During the Middle Permian the basin was transgressed followed by the establishment of a hypersaline carbonate platform associated with deposition of carbonates, carbonate conglomerates, dolomites and evaporites with subordinate isolated siliciclastic beds (e.g. Stemmerik, 2001).
A prominent regional karstification surface developed in the Middle Permian and in the Late Permian an open marine carbonate platform developed on the karstified surface of the Early Permian carbonate platform. On the palaeotopographic highs along the basin margin build-ups developed. Elsewhere, carbonate deposition took place during falling sea-level and thin, laterally extensive units of shallow-marine grainstones rest directly on top of deeper marine shales (Stemmerik, 2001). During lowstands shallow and deep marine sheet sandstones were deposited (Kreiner-Møller & Stemmerik, 2001; GEUS in-house data). These regressive events were probably controlled by tectonic uplift in the hinterland prior to the Early Triassic rifting. During the Late Permian the basin was fluctuating between oxic and anoxic conditions and an important marine source rock was deposited (Fig. 4).
A new rift event took place in the Early Triassic and was associated with a change in stress-system which resulted in NE–SW trending rift faults (Guarnieri et al., 2017). During the syn-rift phase large amounts of sandstone and conglomerate interbedded with marine and terrestrial mudstone were deposited in at least five separate sub-basins (Seidler et al., 2004). Rifting continued into the Middle Triassic with deposition of alluvial fan conglomerates and floodplain sandstones (Fig. 4).
During the Late Triassic post-rift sagging phase continued uniform deposition took place in the Jameson Land and Traill Ø sub-basin (Fig. 4). Late Triassic deposition reflects the northward drift of Pangea and the associated change in climate (Clemmensen 1980a, b) with a gradual transition from sabkha and ephemeral lake sedimentation to shallow, perennial to ephemeral lake and finally to deep perennial lake sedimentation (Fig. 4; Clemmensen 1980a, b; Dam & Surlyk, 1993). The latest Triassic – earliest Jurassic perennial lacustrine deposits are confined to the Jameson Land Basin.
A marine inundation took place in Pliensbachian and a large shallow marine embayment was established in the Jameson Land Basin (Fig. 4; e.g. Dam & Surlyk, 1998; Surlyk et al., 2021). Several potential reservoir units equivalent to some of the most important reservoir units of the mid-Norwegian continental shelf are present in this succession interbedded with intra-formational Toarcian – lowermost Aalenian mudstone seals (Fig. 4).
Onset of Jurassic–Cretaceous rifting took place in late Bajocian. Rifting was intensified during the Bathonian–Kimmeridgian and culminated in the Volgian – early Ryazanian with waning in the late Ryazanian – Hauterivian (Albian) (Fig. 4; e.g. Surlyk, 2003; Surlyk et al., 2021) and resulted in a complete reorganisation of the basin configuration and drainage patterns. During this phase the East Greenland Rift System was gradually transgressed from the south and the deposition expanded far towards the north (Fig. 4; Surlyk, 2003; Surlyk et al. 2021).
The rift system was divided into a series of N–S directed grabens and half-grabens. Only minor faulting appears to have taken place in the Jameson Land Basin and it does not appear to have been significantly affected by this Late Jurassic – Early Cretaceous rift phase, suggesting that south of Kong Oscar Fjord rifting was focused east of Liverpool Land (the present offshore areas).
In the southern part of the East Greenland Rift System, the upper Bajocian – Kimmeridgian succession consists of stepwise backstepping units starting with shallow marine sandstones and ending with relatively deep marine mudstones in some places with sandy gravity-flow deposits and injectites. In the Jameson Land and Milne Land Basins, the uppermost Jurassic – lowermost Cretaceous (Volgian–Ryazanian) succession consists of forestepping, stacked shelf-margin sandstone bodies with associated slope and basinal mudstones and massflow sandstones.
North of Jameson Land, block-faulting and tilting began in the late Bajocian and culminated in the middle Volgian with formation of strongly tilted fault blocks (Fig. 4). The succession records continued stepwise deepening with formation of Upper Jurassic rift-climax strata reaching thicknesses of several kilometres. The Volgian is represented by a thick wedge of deep-water conglomerates and pebbly sandstones passing basinwards into mudstones deposited in fault-attached slope aprons and coalescing submarine fans. Several potential reservoir units equivalent to some of the most important reservoir units of the mid-Norwegian continental shelf are present in this succession together with a world-class Oxfordian – Rhyazanian marine source rock was deposited throughout the AU5 area (Fig. 4).
Continued phases of widespread rifting took place during the Late Cretaceous controlling sedimentation in the East Greenland Rift System, mainly in a deep-marine setting (Fig. 4). A major fall in relative sea-level took place in the Turonian and resulted in the establishment of coarse-grained basin floor fan systems that persisted through the Late Cretaceous (e.g. Hovikoski et al. 2012; Bjerager et al. 2020). Continued phases of widespread rifting took place prior to break-up culminating with widespread Ypresian uplift, voluminous volcanism and subaerial exposure.
Onset of uplift took place around the Selandian (~60 Ma; Japsen et al., 2021). During Danian–Thanetian coarse-grained gravity flow deposits were intercalated with offshore basinal mudstones. Faulting, block rotation and hinterland uplift took place along entire East Greenland margin. During the Paleocene-Eocene transition rapid fluvio-lacustrine sedimentation and uplift occurred associated with extrusion of subaerial lavas across the sea way. This eventually culminated with continental break-up and sea floor spreading from 54 Ma and onwards (Hovikoski et al. 2021).
Plate reconstruction by Gaina et al. (2017) show that the North Greenland Sea was almost closed by a volcanic ridge at least until ~53 Ma (Hovikoski et al. 2021). A narrow, shallow water strait may have existed between Greenland and Europe. Volcanism continued locally into the Miocene and the East Greenland rifted margin turned into a passive margin.
Off Liverpool Land and Blosseville Coast rifting continued following the initial break-up of the North Atlantic and gave rise to the Scoresby Basin (Fyhn et al., in press) that concluded the latest Oligocene or early Miocene continental break-up of the Jan Mayen microcontinent and East Greenland. The basin contains several kilosmetres of Eocene – lower Miocene fan-delta deposits that accumulated during downfaulting along the East Greenland Escarpment and which further seawards intercalate with basalts. The fan-delta deposits rest on Paleocene basalts that most probably cover Palaeozoic–Mesozoic strata.
Plays and Play Elements
Based on seismic interpretation, well and outcrop data, 12 plays have been established in central East Greenland (Fig. 4). However, only Plays 9 and 7 (Permian and Upper Jurassic–Lower Cretaceous) are included in the Yet-to-Find evaluation since all other plays are not considered to have any exploration potential in the area. Play 7 has been split in two for modelling purposes since this play includes two source rock units that are separate in both space and time. Hence, modelling includes uppermost Triassic – Lower Jurassic lacustrine source rocks in the Jameson Land region and Upper Jurassic – Lower Cretaceous marine shales elsewhere. Ten source rock intervals have been documented or inferred in the onshore and reservoir rocks are present at all stratigraphic levels.
Petroleum Systems
Onshore indications of viable petroleum systems in AU5 include a variety of examples of oil-staining/seepage recorded in both fully cored boreholes and in outcrops (Christiansen & Bojesen-Koefoed, 2021). These various occurrences can be classified as (1) associated with a mature source rock in immediate vicinity to the stain; (2) migrated, i.e. not associated with a known nearby source rock; (3) associated with mineralisations; (4) gas seeps (see link to GIS compilation in Christiansen & Bojesen-Koefoed (2021) for locations and references). Source-associated stains generated from the Middle Devonian are found at Ankerbjergselv; from the Permian at Kap Stosch, Margrethedal and Wegener Halvø; from the Carnian at Mols Bjerge; from the Upper Jurassic in the Sjællandselv and Blokelv boreholes; from the Cretaceous in the Nanok-1 borehole and in outcrops near to the wellsite (Bojesen-Koefoed et al., 20209. Migrated oil traces that cannot be readily associated with a local source are found in Tobiasdal, Savoia Halvø, Kap Dalton and in the so-called exhumed oilfields on Traill Ø and Geographical Society Ø. Mineralisation-associated bitumen, so-called carburan, is found Randbøldal and gas seeps are found in Coloradodal and Rømerfjord. Only sparse information is available from the offshore areas, but a remote sensing study by Vis et al. (2017), using synthetic aperture radar (SAR) suggests seepage in Kong Oscar Fjord and in two areas located wholly or partly east of the continent–ocean boundary.
Potential petroleum source rocks in AU5 include a total of ten units, but five of these are not considered due to their nature, level of documentation or areal distribution. The source rocks considered for AU5 are listed in Table 1 and discussed below.
Devonian source rocks (Play 12) include equivalents of the highly prolific Upper Devonian lacustrine shales of the Obrutschew Bjerg Formation, known from the Ymer Ø – Gauss Halvø region south of AU4 (Christiansen et al., 1990a, 1992; Olsen 1993; Olsen & Larsen 1993). This unit has not been included in the Yet-to-Find evaluation due to its restricted volume and areal distribution. However, where present it may constitute an important supplementary source of petroleum.
The Carboniferous (Play 10) is represented by the Upper Carboniferous lacustrine deposits of the Traill Ø Group (Christiansen et al. 1990a, 1992; Piasecki et al. 1990; Stemmerik et al. 1990, 1993). The Upper Carboniferous shales of the Traill Ø Group deposits are widespread in AU5 and sometimes include thick and highly prolific lacustrine source rocks. This unit has not been included in the Yet-to-Find evaluation due to its restricted volume and areal distribution. However, where present it may constitute an important supplementary source of petroleum.
Upper Permian (Play 9) source rocks of the Ravnefjeld Formation are widespread and well-documented in AU5 (Christiansen et al. 1990b, 1993; Piasecki & Stemmerik 1991). The succession includes two prolific marine shale intervals with a combined thickness of c. 15 m, sandwiched between non-source mudstones.
Play 7 includes two different source rock units, separate in age and areal distribution. In the Jameson Land Basin and offshore areas south hereof, lacustrine shales and coals of the Kap Stewart Group form prolific petroleum source rocks that are absent from other parts of AU5, where Upper Jurassic – Lower Cretaceous marine shales constitute the source rock of Play 7. In accordance with this the Yet-to-Find evaluation for Play 7 is split in two.
Uppermost Triassic – Lowermost Jurassic (Play 7) Kap Stewart Group lacustrine shales and coals constitute prolific source rocks in the Jameson Land Basin. The succession has been thoroughly documented by Dam & Christiansen (1990), Christiansen et al. (1992), Dam & Surlyk (1992, 1993) and Surlyk (2003).
Upper Jurassic – Lower Cretaceous (Play 7) petroleum source rocks, equivalents of the principal source rocks of the greater North Sea Petroleum province and the Barents Sea, are widely distributed and exposed in North-East Greenland, and the presence of prolific basinal facies deposits in part of the offshore areas of AU5 can reasonably be assumed. Their properties are estimated from well-studied successions onshore North-East Greenland (Requejo et al. 1989, Christiansen et al. 1992, Surlyk & Noe-Nygaard 2001; Surlyk 2003; Strogen et al. 2005; Bojesen-Koefoed et al. 2018; Ineson & Bojesen-Koefoed 2018).
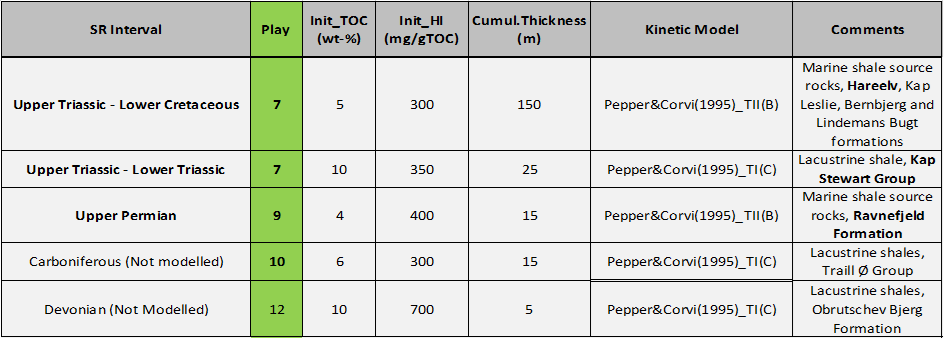
Table 1 – Source rock intervals identified for Assessment Unit 5 showing values selected for the basin modelling study.
Basin Modelling
In the conducted basin modelling study, the potential petroleum source rocks data listed in Table 1 have been used as source rock characteristics. Important input considerations such as amount of erosion, has been summed up to one post- with a uniform erosion of 2 km onshore Jameson Land and decreasing to below 300 m in the offshore Scoreby Sund Basin. The amount of uplift is based on measured vitrinite reflection data from onshore Jameson Land Basin wells and interpretation of seismic data (Scoreby Sund Basin). The lack of deep wells impedes calibration of the maturity model. An averaged low heat flow history (35 mW/m2) with minor Permian rifting peak (45 mW/m2) and a volcanic peak of 55 mW/m2 was used. A simple uniform heat flow history of 55 mW/m2 was used for the Scoresby Sund Basin.
In the Jameson Land Basin thickness of Upper Cretaceous sediments and Paleocene volcanic rocks, as wells as enhanced heat flow during the Paleocene, are particular important factors for the thermal maturation of the source rocks. As the Jameson Land Basin was uplifted and eroded after the short period of volcanism temperature decreased gradually resulting in a decrease or cessation of hydrocarbon expulsion from source rock intervals.
The basin modelling results show that the Upper Permian Ravnefjeld source rock (Play 9 see Table 1) and older source rock successions are post-mature over most of the Jameson Land Basin. The Lower Jurassic Kap Stewart source rock interval (Play 7 see Table 1) is in the oil window over large parts of the basin except in the southwestern area where it has entered the late oil–gas window. The Upper Jurassic Hareelv source rock interval (Play 7 see Table 1) is in immature over large parts of the basin except in local areas where it has entered the early oil window. In the Scoresby Sund Basin, most of potential source rock intervals below the basalt are post-mature assuming a 2000 m thick basaltic cover.
An important element of the charge modelling is the large amount of post-volcanic uplift, where the hydrocarbons charge is switched off. Likewise, local timing risk issues must be addressed to fully assess the prospectivity of specific areas.
Assessment Process
The assessment process for the resource evaluation of AU4 is summarised in the “Yet-To-Find Triangle” shown in Figure 6. Basin evaluation is the basic activity describing the structural and stratigraphic framework leading into construction of Gross Depositional Environment (GDE) maps and identification of source rocks, reservoirs and regional seals that form the basis for definition of the plays. IES PetroMod v16.2 has been used to compile and setup a 3D basin reference model to assess maturity and possible migration for expected source rock intervals.
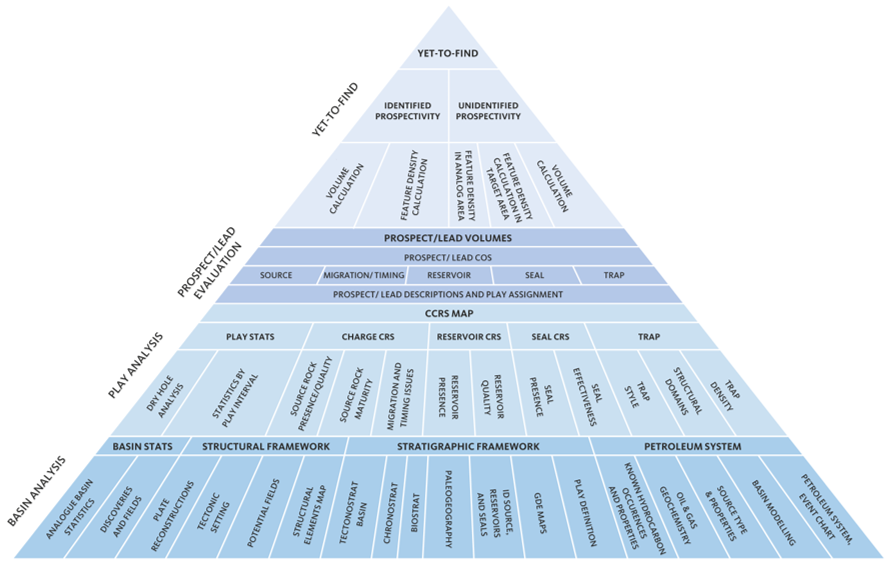
Figure 6 – Resource evaluation workflow used in the Greenland Resource Assessment.
The results from the Basin Evaluation feed into the Play Analysis. In this analysis, numeric Common Risk Segment (CRS) and Composite Common Risk Segment (CCRS) maps have been constructed based on all available geological and geophysical data and exploration history data.
The following play risk elements have been assessed: Reservoir Presence (based on GDE maps), Reservoir Effectiveness (based on depth to interval maps), Seal Effectiveness (based on overburden thickness maps corrected for uplift) and Charge-Migration (based on source presence and maturity maps and drainage maps), and Trap Presence (based on possibility to obtain high-quality seismic data).
Since the Permian Ravnefjeld Formation and the Jurassic Kap Stewart and Hareelv Formations are regarded to be only important source rock intervals in AU5, a single-charge scenario is applied for all plays. A detailed description of the charge evaluation process is found in the PowerPoint presentations available on the data portal.
Volumes for the four mapped leads mapped by NUNAOIL have been integrated into the play evaluation and the identified prospectivity has been calculated. For the volume calculations of individual leads the oil-water contact has been picked halfway between the top of the structure and spill point, since source rock quality analysis does not support fill-to-spill scenarios.
Gas risk has not been assessed and all volumes are in MMBOE. For analysis on volumetrics of individual phases, more detailed analysis and mapping must be carried out.
The Yet-to-Find analysis is based on a feature (lead) density calculation approach for each of the identified play intervals. In Assessment Unit 5 estimates of Analog Feature Density for each play is based on observed analogue feature densities obtained from well-explored areas in Assessment Area 4 (North-East Greenland) which show a similar structural development as the areas in question in AU5. Based on these estimates the unidentified prospectivity has been calculated for the AU5.
Resource Assessment
The resource assessment provides an estimate of the play-based Yet-to-Find potential of conventional hydrocarbons. The assessment includes Plays 6, 7 and 9 (Fig. 4). Plays 1 and 2 are located at very shallow depths below seabed without any known possible source rocks and are therefore not considered prospective. The deeper Devonian, Carboniferous, Triassic, Late Cretaceous, latest Cretaceous – Paleocene and Eocene plays have all been fully analysed but are excluded from the Yet-to-Find assessment since prospectivity of these plays is very low due to various reasons such as very deep burial, lack of regional source, lack of regional seal or severe charge issues.
Known Prospectivity
The first step in the Yet-to-Find Assessment was to make Gross-Rock Volumes for the leads mapped by NUNAOIL in AU5. Only seven leads on Carboniferous and Permian level have been recognised and evaluated in Assessment Unit 5 (Fig. 7). Gross-Rock Volume calculations were made in Player® based on area-depth pairs from the top of structure to spill-point. Area-depth pairs were derived from either mapping of the structures in Petrel or digitizing of closure and contour lines in ArcGIS from company
reports.
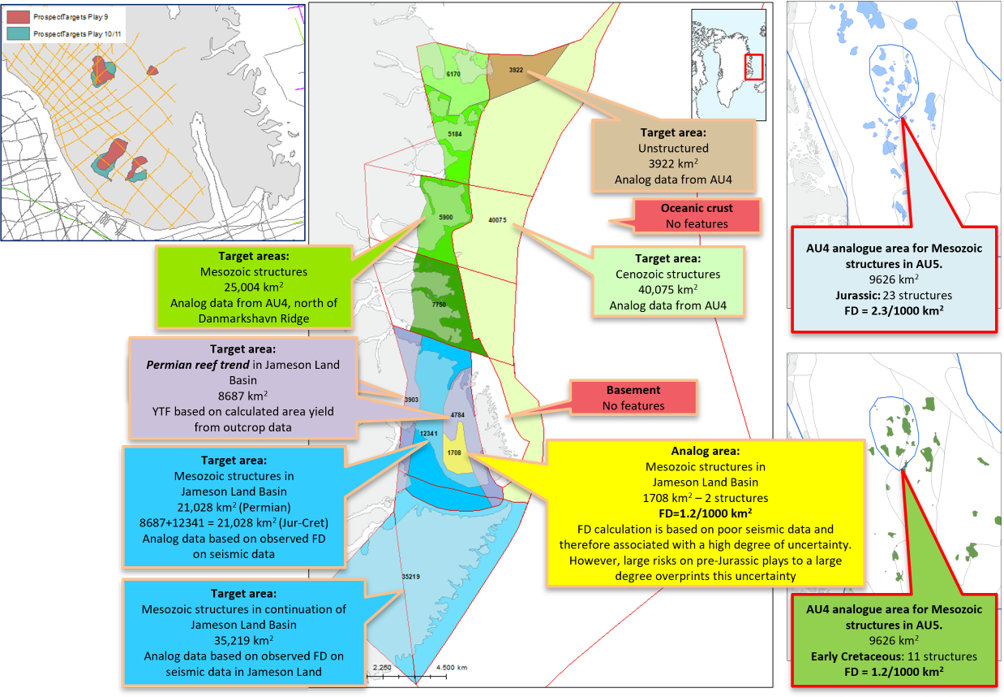
Figure 7 – Analogue areas in AU4 and AU5 and target areas in AU5 for the Yet-to-Find calculations and Identified leads on Permian and Carboniferous level based on seismic mapping.
For the hydrocarbon volume calculations, the following input parameters have been considered for all leads: 1) Porosity, 2) Gross and net sand thicknesses and Net-to-Gross Ratio, 3) Oil Saturation, 4) Oil formation volume factor (Bo), 5) Recovery Factor (RF), and 6) Gas expansion factor (E). These input parameters are based mainly on outcrop and stratigraphic borehole information.
The known prospectivity appears from integrating the calculated identified lead volumes (see section above) with the CCRS maps providing the risked volumes for the leads.
Unidentified Prospectivity
As mentioned above, the unidentified prospectivity analysis is based on the observed Feature Densities (FD) in analogue areas in AU4 with good seismic coverage. Areas composed of oceanic crust or basement rocks are not included as Target Areas (Fig. 7). The prospectivity of the Permian reef trend in eastern Jameson Land is based on area yield calculations derived from reservoir volume calculations of reefs exposed on Wegener Halvø (Stemmerik 1991).
The “Future Target Area FD (per play)” = “Analog Area (FD)” ÷ “Identified Target Area FD”.
The unidentified prospectivity is the “Future Target Area FD (per play)” × “Mean volume per Feature MMBOE)”.
A summary of the results by Area of Interest for Identified Prospectivity and Unidentified Prospectivity is shown in Figure 8.
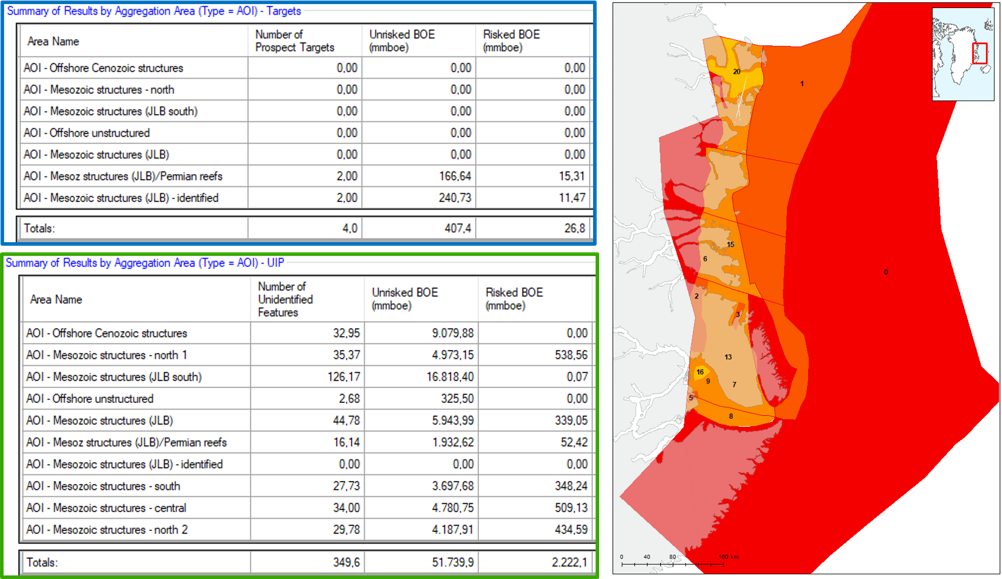
Figure 8 – Summaries of Identified and Unidentified Prospectivity in Assessment Unit 5. Map shown Total Risk maximum risk value stack of Plays 3–9. The Total Risked Recoverable (Mean Case) is 2,350 MMBOE including volumes from Permian reef trend in eastern Jameson Land.
Portfolio Analysis
Although Total Risk appears to be acceptable for large parts of onshore AU5 (Fig. 8) it should be noticed that these risks rely on the possibility to acquire high-quality geophysical data in the entire onshore region or that detailed geological mapping will be able to define valid subsurface structures.
Furthermore, phase risk (gas) has not been dealt with here as well as local timing issues that must be addressed to fully assess the prospectivity of specific areas.
The most prospective areas are found the Mesozoic basins north of the Jameson Land Basin where area yields of ~85 MMBOE/1000 km2 are calculated (Fig. 9).
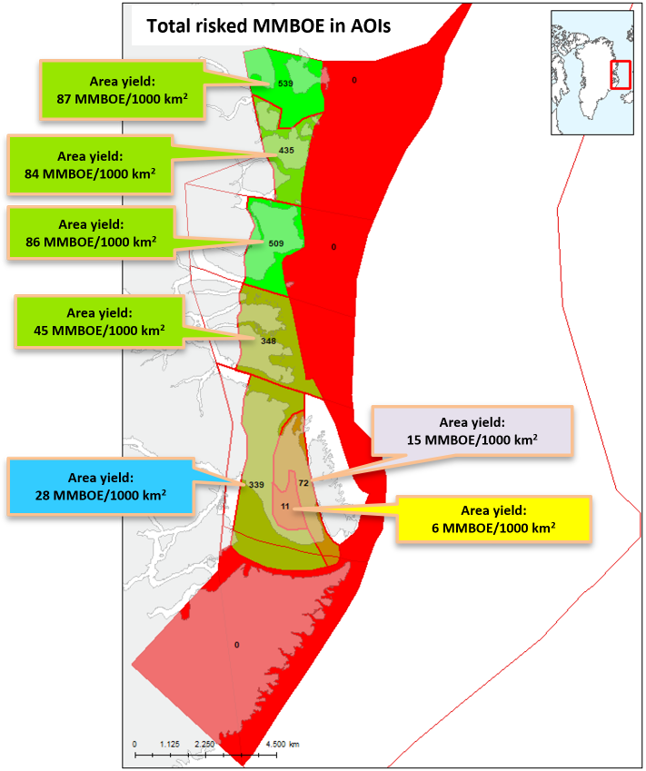
Figure 9 – Total risked MMBOE in Areas of Interest (AOI) in AU5.
Deliverables
The results from this study including a Player® ArcGIS project and an extensive slide pack are available for the petroleum exploration industry by contacting Thomas Varming at NUNAOIL.
References
Bjerager, M. et al., 2020: Cretaceous lithostratigraphy of North-East Greenland. Bulletin of the Geological Society of Denmark 68, 37–93. doi.org/10.37570/bgsd-2020-68-04
Bojesen-Koefoed, J.A., Bjerager, M., Nytoft, H.P., Petersen, H.I., Piasecki, S. & Pilgaard, A. 2018: Petroleum potential of the Upper Jurassic Hareelv Formation, Jameson Land, East Greenland. In: Ineson & Bojesen-Koefoed (eds) Geological Survey of Denmark and Greenland Bulletin 42, 85–113. doi.org/10.34194/geusb.v42.4314
Bojesen-Koefoed, J.A., Alsen, P., Bjerager, M., Hovikoski, J., Ineson, J., Nytoft, H.P., Nøhr-Hansen, H., Petersen, H.I., Pilgaard, A. & Vosgerau, H., 2020: A mid-Cretaceous petroleum source-rock in the North Atlantic region? Implications of the Nanok-1 fully cored borehole, Hold with Hope, North-East Greenland Marine and Petroleum Geology 117. doi.org/10.1016/j.marpetgeo.2020.104414
Christiansen, F.G. & Bojesen-Koefoed, J.A., 2021: Inventory of onshore petroleum seeps and stains in Greenland: a web-based GIS model, GEUS Bulletin 47, 20 pp. doi.org/10.34194/geusb.v47.6519
Christiansen, F.G., Olsen, H., Piasecki, S. & Stemmerik, L., 1990a: Organic geochemistry of Upper Palaeozoic lacustrine shales in the East Greenland basin. Organic Geochemistry 16, 287–294.
Christiansen, F.G., Piasecki, S. & Stemmerik, L,. 1990b: Thermal maturation history of the Upper Permian succession in the Wegener Halvø area, East Greenland. Rapport Grønlands Geologiske Undersøgelse 148, 109-114. doi.org/10.34194/rapggu.v148.8129
Christiansen, F. G., Dam, G., Piasecki, S. & Stemmerik, L., 1992: A review of Upper Palaeozoic and Mesozoic source rocks from onshore East Greenland.: Generation, accumulation and production of Europe`s hydrocarbons II. In: Spencer, A. M. (ed.), Special Publication of the European Association Petroleum Geologists 2, 151–161.
Christiansen, F.G., Piasecki, S., Stemmerik, L. & Telnæs, N., 1993: Depositional environment and organic geochemistry of the Upper Permian Ravnefjeld Formation source rock in East Greenland. American Association of Petroleum Geologists Bulletin 77, 1519-1537.
Clemmensen, L., 1980a: Triassic rift sedimentation and palaeogeography of central East Greenland. Grønlands Geologiske Undersøgelse Bulletin 136, 72 pp. doi.org/10.34194/bullggu.v136.6678
Clemmensen, L., 1980b: Triassic lithostratigraphy of East Greenland between Scoresby Sund and Kejser Franz Joseph Fjord. Grønlands Geologiske Undersøgelse Bulletin 139, 56 pp. doi.org/10.34194/bullggu.v139.6681
Dam, G. & Christiansen, F. G., 1990. Organic geochemistry and source potential of the lacustrine shales of the Upper Triassic – Lower Jurassic Kap Stewart Formation, East Greenland. Marine and Petroleum Geology 7, 428–443. doi.org/10.1016/0264-8172(90)90019-D
Dam, G. & Surlyk, F., 1993: Cyclic sedimentation in a large wave and storm-dominated anoxic lake; Kap Stewart Formation (Rhaetian–Sinemurian), Jameson Land, East Greenland. In: Posamentier et al. (eds): Sequence stratigraphy and facies associations. International Association of Sedimentologists Special Publication 18, 419–448.
Dam, G. & Surlyk, F., 1998: Stratigraphy of the Neill Klinter Group; a Lower – lower Middle Jurassic tidal embayment succession, Jameson Land, East Greenland. Geology of Greenland Survey Bulletin 175, 80 pp. doi.org/10.34194/ggub.v175.5026
Fyhn, M. B. W. & Hopper, J. R., in press: NE Greenland Composite Tectono-Sedimentary Element, northern Greenland Sea and Fram Strait. In: Drachev, S. S., Brekke, H., Henriksen, E. and Moore, T. (eds): Sedimentary successions of the Arctic region and their hydrocarbon prospectivity. Geological Society, London, Memoirs 57.
Fyhn, M.B.W., Hopper, J.R., Sandrin, A., Lauridsen, B.W., Heincke, B.H., Nøhr-Hansen, H., Andersen, M.S., Alsen, P. & Nielsen, T., 2021: Three-phased latest Jurassic–Eocene rifting and mild mid-Cenozoic compression offshore NE Greenland. Tectonophysics 815, 228990. doi.org/10.1016/j.tecto.2021.228990
Fyhn, M. B. W., et al., in press: North-East and central East Greenland Rifted Tectono-Sedimentary Element. In: Drachev, S. S., Brekke, H., Henriksen, E. and Moore, T. (eds): Sedimentary Successions of the Arctic Region and their Hydrocarbon Prospectivity. Geological Society, London, Memoirs 57.
Gaina, C., Nasuti, A., Kimbell, G.S. & Blischke, A. 2017: Break-up and seafloor spreading domains in the NE Atlantic. Geological Society, London, Special Publications 447, 393–417. doi.org/10.1144/SP447.12
Gautier, D.L., 2017: Geology and assessment of undiscovered oil and gas resources of the East Greenland Rift Basins Province, 2008. In: Moore, T.E. & Gautier, D.L. (eds): The 2008 Circum-Arctic Resource Appraisal, Chapter K. U.S. Geological Survey Professional Paper 1824. doi.org/10.3133/pp1824K
Guarnieri, P., Brethes, A. & Rasmussen, T., 2017: Geometry and kinematics of the Triassic rift basin in Jameson Land (East Greenland). Tectonics 36, 1-13. doi.org/10.1002/2016TC004419
Hovikoski, J et al., 2012: The Cretaceous of NE Greenland: Sequence stratigraphy (Subproject 6). Danmarks og Grønlands Geologiske Undersøgelse Rapport 2012/53, 71 pp.
Hovikoski, J. et al. 2021: Fragmentation of Norwegian–Greenland Seaway during the Early Paleogene. Communications Earth & Environment 2, 172. doi.org/10.1038/s43247-021-00249-w
Ineson, J.R. & Bojesen-Koefoed J.A. (eds) 2018: Petroleum geology of the Upper Jurassic – Lower Cretaceous of East and North-East Greenland: Blokelv-1 borehole, Jameson Land Basin. Geological Survey of Denmark and Greenland Bulletin 42, 168 pp.
Ineson, J. R. et al., 2020: Regional impact of Early Cretaceous tectono-magmatic uplift in the Arctic: Implications of new data from eastern North Greenland. Terra Nova 2020, 1–9. doi.org/10.1111/ter.12514
Japsen, P., Green, P. F., Bonow, J. M., Bjerager, M. & Hopper, J. R., 2021: Episodic burial and exhumation in North-East Greenland before and after opening of the North-East Atlantic. GEUS Bulletin 45(2), 154 pp. doi.org/10.34194/geusb.v45.5299
Kreiner-Møller, M. & Stemmerik, L. 2001: Upper Permian Iowstand fans of the Bredehorn Member, Schuchert Dal Formation, East Greenland. In: Martinsen, O. J. and Dreyer, T.: Sedimentary Environments Offshore Norway – Palaeozoic to Recent. NPF Special Publication 10, 51–65. doi.org/10.1016/S0928-8937(01)80008-4
Larsen, P.-H. & Bengaard, H.-J., 1991: Devonian basin initiation in East Greenland: a result of sinistral wrench faulting and Caledonian extensional collapse. Journal of the Geological Society, London, 148, 355-368. doi.org/10.1144/gsjgs.148.2.0355
Olsen, H., 1993: Sedimentary basin analysis of the continental Devonian basin in North-East Greenland. Bulletin Grønlands Geologiske Undersøgelse 168, 80 pp. doi.org/10.34194/bullggu.v168.6724
Olsen, H. & Larsen, P. H., 1993: Lithostratigraphy of the continental Devonian sediments in North-East Greenland. Bulletin Grønlands Geologiske Undersøgelse 165, 108 pp. doi.org/10.34194/bullggu.v168.6724
Piasecki, S., Christiansen, F. G. & Stemmerik, L., 1990: Depositional history of a Late Carboniferous organic-rich shale from East Greenland. Bulletin of Canadian Petroleum Geology 38, 273-287. doi.org/10.1016/B978-0-444-88943-0.50009-5
Piasecki, S. & Stemmerik, L., 1991: Late Permian anoxia of central East Greenland. In: Tyson, R. V. & Pearson, T. H. (eds): Modern and ancient shelf anoxia. Geological Society London Special Publication 58, 275-290. doi.org/10.1144/GSL.SP.1991.058.01.18
Requejo, A. G., Hollywood, J., & Halpern, H. I., 1989: Recognition and source correlation of migrated hydrocarbons in Upper Jurassic Hareelv Formation, Jameson Land, East Greenland. American Association of Petroleum Geologists Bulletin 73, 1065-1088. doi.org/10.1306/44B4A53C-170A-11D7-8645000102C1865D
Seidler, L. Steel, R. J., Stemmerik, L. & Surlyk, F., 2004. North Atlantic marine rifting in the Early Triassic: new evidence from East Greenland. Journal of the Geological Society 161, 583–592.
doi.org/10.1144/0016-764903-063
Stemmerik, L., 1991: Reservoir evaluation of Permian buildups in the Jameson Land basin, East Greenland. Rapport Grønlands Geologiske Undersøgelse 149, 28 pp. https://geusjournals.org/index.php/rapggu/article/view/8134/14004
Stemmerik, L., 2001: Sequence stratigraphy of a low productivity carbonate platform succession: the Upper Permian Wegener Halvø Formation, Karstryggen Area, East Greenland. Sedimentology 48, 79–97.
Stemmerik, L., Christiansen, F. G. & Piasecki, S., 1990: Carboniferous lacustrine shale in East Greenland – additional source rock in the northern North Atlantic? In: Katz, B. J., (ed.): Lacustrine basin exploration – case studies and modern analogs, American Association of Petroleum Geologists Memoir 50, 277–286.
doi.org/10.1306/M50523C17
Stemmerik, L., Christiansen, F. G., Piasecki, S., Jordt, B., Marcussen, C. & Nøhr-Hansen, H., 1993: Depositional history and petroleum geology of the Carboniferous to Cretaceous sediments in the northern part of East Greenland. In: Vorren, T.O. et al. (eds): Arctic geology and petroleum potential, NPF Special Publication 2, 67–87. doi.org/10.1016/B978-0-444-88943-0.50009-5
Strogen, D. P., Burwood, R. & Whitham, A. G., 2005: Sedimentology and geochemistry of Late Jurassic organic-rich shelfal mudstones from East Greenland: regional and stratigraphic variations in source rock quality. In: Doré, A. G, & Vining, B. A. (eds): Petroleum Geology: Northwest Europe and Global Perspectives – Proceedings of the 6th Petroleum Geology Conference, 903–912. Geol. Soc. London. doi.org/10.1144/0060903
Surlyk, F., 1978: Jurassic basin evolution of East Greenland. Nature 274(5667), 130–133. doi.org/10.1038/274130a0
Surlyk, F. 2003: The Jurassic of East Greenland: a sedimentary record of thermal subsidence, onset and culmination of rifting. In: Ineson, J,R. & Surlyk F. (eds): The Jurassic of Denmark and Greenland. Geological Survey of Denmark and Greenland Bulletin 1, 659-722. doi.org/10.34194/geusb.v1.4674
Surlyk, F. & Noe-Nygaard, N., 2001: Sand remobilisation and intrusion in the Upper Jurassic Hareelv Formation of East Greenland. Bulletin of the Geological Society of Denmark 48, 169-188. doi.org/10.37570/bgsd-2001-48-10
Surlyk, F. et al., 2021: Jurassic stratigraphy of East Greenland. GEUS Bulletin 46, 116 pp. doi.org/10.34194/geusb.v46.6521
Vigran J. O. S., Stemmerik, L. & Piasecki, S., 1999: Stratigraphy and depositional evolution of the uppermost Devonian‐carboniferous (Tournaisian‐Westphalian) non‐marine deposits in north‐east Greenland. Palynology 23(1), 115-152. doi.org/10.1080/01916122.1999.9989525
Vis, G.-J., 2017. Geology and seepage in the NE Atlantic region. In: Péron-Pinvidic, G. et al. (eds) 2017. The NE Atlantic Region: A Reappraisal of Crustal Structure, Tectonostratigraphy and Magmatic Evolution. Geological Society, London, Special Publications 447, 443–455. https://doi.org/10.1144/SP447.16
Contents
- Executive Summary
- Introduction
- Database
- Structural and Stratigraphic Framework
- Analogue Basins – West of Shetland and Ireland
- Petroleum Systems
- Assessment Process
- Resource Assessment
- Deliverables
- References